Challenges and opportunities for treating intrahepatic cholangiocarcinoma: targeted therapy
Abstract
Over the last two decades, substantial progress has been made in the scope of molecular targeted therapy, leading to transformative advancements in the treatment of various malignancies, including biliary tract cancer (BTC). BTC represents a heterogeneous group of aggressive tumors with historically poor prognoses. However, recent discoveries of novel molecular alterations in BTC have provided new avenues for targeted therapeutic interventions, exemplified by the approval of pemigatinib, specifically designed for FGFR2 gene fusions or rearrangements in advanced BTC. Furthermore, subsequent regulatory approvals and ongoing clinical trials focusing on specific gene mutations have considerably expanded the array of treatment options available, augmenting the potential for personalized treatment strategies. In light of these developments, this review aims to furnish a comprehensive and up-to-date account of the molecular characteristics and potential targeted therapies in BTC. By presenting insights into novel therapeutic approaches and outlining prospective directions for translational and clinical investigations, this review seeks to contribute to the ongoing progress and optimization of therapeutic approaches in managing BTC.
Keywords
INTRODUCTION
Biliary tract cancer (BTC) is a heterogeneous group of biliary cancers that occur at various locations along the biliary tree, representing approximately 3% of gastrointestinal malignancies. BTC is divided into several main subtypes based on its location: intrahepatic cholangiocarcinoma (iCCA), perihilar cholangiocarcinoma (pCCA), and distal cholangiocarcinoma (dCCA). These subtypes represent different locations along the biliary tree and contribute to the heterogeneity of BTC [Figure 1]. The incidence of BTC is steadily increasing worldwide[1]. Unfortunately, the prognosis for BTC remains grim due to delayed detection and the limited number of patients (approximately 30% or less) who qualify for curative surgery[2]. Furthermore, while several study results support the use of neoadjuvant therapy to potentially enhance survival and achieve a higher R0 resection rate, there is no unanimous consensus on its necessity[3]. Additionally, most patients are diagnosed at advanced stages with distant metastasis and extensive liver involvement. The rarity of the disease and the lack of effective treatments intensify the urgency to explore novel strategies for managing this devastating condition. The treatment of advanced BTC poses a significant challenge because the condition remains incurable and alternative treatment options are limited. As a result, the current approach focuses primarily on supportive care and systemic therapy to improve overall survival (OS) and enhance quality of life. Despite efforts, the prognosis for advanced BTC patients varies considerably, with reported median OS ranging from 4 to 12 months in different studies[4-6]. Therefore, the ongoing challenges in treating advanced or recurrent BTC underscore the necessity for further advancements in therapeutic interventions.
Figure 1. Illustration of the anatomical sites of BTC includes intrahepatic cholangiocarcinoma (iCCA), perihilar cholangiocarcinoma (pCCA), and distal cholangiocarcinoma (dCCA). iCCA refers to malignancies located in the periphery of the second-order bile ducts, while pCCA originates in the right or left hepatic duct and/or at their junction. On the other hand, dCCA involves the common bile duct. Each subtype of BTC occupies a distinct anatomical position within the biliary tract. The collective term extrahepatic cholangiocarcinoma (eCCA) is used to encompass both pCCA and dCCA.
The gemcitabine and cisplatin combination regimen has long been the standard systemic therapy for advanced BTC, based on the findings of the ABC-02 study[6]. This phase 3 trial compared the efficacy of gemcitabine alone versus gemcitabine plus cisplatin and showed better OS and progression-free survival (PFS) with the combined therapy. Patients received gemcitabine plus cisplatin for approximately 8 cycles, followed by close surveillance. It also showed a better response rate (RR) in the combination regimen group than in the gemcitabine monotherapy group (26.1 % and 15.5 %, respectively). Subgroup analysis revealed the benefits of the combination therapy across different tumor locations, including intrahepatic, extrahepatic, and gallbladder. These findings established the gemcitabine and cisplatin therapy as the standard systemic treatment for advanced BTC.
In the pursuit of enhancing treatment outcomes, the TOPAZ-1 study investigated the addition of immunotherapy to chemotherapy for advanced BTC[7]. The study included patients who received up to 8 cycles of gemcitabine/cisplatin plus durvalumab, followed by durvalumab maintenance, compared to those who received gemcitabine/cisplatin followed by placebo. The results demonstrated a positive outcome with durvalumab, showing a median OS of 12.8 months compared to 11.5 months with placebo (hazard ratio [HR] of 0.80; 95% confidence interval [CI]: 0.66-0.97 months; P = 0.021). The study also showed a higher RR in the durvalumab group compared to the placebo group [26.7% and 18.7%, respectively, odds ratio of 1.60, (95%CI: 1.11-2.31)]. Subgroup analyses based on sex, PD-L1 expression, tumor location (intrahepatic vs. extrahepatic or gallbladder), and Asian vs. non-Asian populations all showed benefits from the combination therapy. Additionally, the PFS was improved in durvalumab group. Consequently, the combination of gemcitabine/cisplatin plus durvalumab has emerged as a new frontline standard of treatment for advanced BTC, presenting a promising advancement in treatment options.
Despite the advancements made in systemic treatment over time, the prognosis of the disease remains unfavorable as it continues to worsen despite undergoing initial treatment. Unfortunately, only a limited number of patients with BTC who experience disease progression following their first-line therapy are eligible to undergo second-line chemotherapy, further limiting their treatment opportunities. According to the TOPAZ-1 trial, 42.5% of patients received subsequent anticancer therapy following immune-chemotherapy[7]. There are several factors that contribute to the limitation of advancing beyond the first line of treatment. One such factor is the insufficient remaining healthy liver parenchyma, which significantly restricts the feasibility of receiving cytotoxic systemic treatments, often resulting in poor outcomes. Additionally, the overall performance status of patients with advanced or metastatic BTC tends to be generally poor, further complicating treatment decisions and options. Infection poses another significant barrier to overcome, as advanced BTC is often complicated by cholangitis, sepsis, and other issues associated with the biliary tree.
When patients with BTC experience disease progression on the first line of therapy and are able to proceed to second-line systemic treatment, the decision regarding the subsequent line of therapy becomes complex. This complexity arises from the absence of a well-defined global treatment standard for this stage. The ABC-06 trial has shown improved survival in patients who received second-line therapy with folinic acid, fluorouracil, and oxaliplatin (FOLFOX) in combination with active symptom control (ASC) compared to ASC alone[4]. However, despite these advancements, the efficacy and prognosis remain discouraging, with median OS at 6.2 months with FOLFOX.
Due to the discouraging prognosis and limited treatment options in BTC, advancements in molecular techniques like next-generation sequencing (NGS) have paved the way for alternative approaches. Moreover, patients with BTC display significant variations in their molecular characteristics and genetic abnormalities, which can vary depending on their specific anatomic locations [Table 1]. This diversity in molecular features makes BTC a potential candidate for targeted therapy. As a result, molecular analysis has now become a routine part of diagnostic testing for advanced BTC cases, providing valuable insights for treatment decisions and enhancing treatment efficacy. In the upcoming section, we will delve into diverse targeted therapies for BTC, exploring their effectiveness and research findings in the context of this challenging cancer.
The prevalence of genetic alterations in BTC based on the anatomic sites
Genetic Alteration | Anatomic Site | ||
iCCA | eCCA | GBC | |
IDH1,2 Mutation | 13%-30%[8,9] | 4.7%[10] | - |
FGFR2 Fusion | 10%-15%[11] | - | - |
BRAF V600E Mutation | 1.5%[12] | - | 0.5%[12] |
HER2 Alteration | 2.2%[13] | 7.5%[13] | 12.6%[13] |
KRAS G12C Mutation | 1.2% across all sites[14] | ||
NTRK Fusion | 0.75% across all sites[15] | ||
RET Fusion | 0.15%[16] | 0.11%[16] | - |
MSI-H/dMMR | 2.06%[17] | 4%[10] | 5%[18] |
TMB-H | 4% across all sites[19] |
TARGETED THERAPIES FOR BILIARY TRACT CANCER
Isocitrate dehydrogenase inhibitors
IDH gene mutations are frequently observed in approximately 13%-30% of iCCA cases, establishing them as the most prevalent targetable mutations in BTC[8,9]. Extensive research has focused on the IDH gene in various cancers, particularly acute myeloid leukemia and other myeloid malignancies. Although the exact mechanisms underlying the oncogenic effects of IDH mutations remain unclear, it is believed that these mutations contribute to the excessive accumulation of metabolites associated with cancer development[20]. Specifically, IDH1 mutations have been shown to enhance the conversion of α-ketoglutarate to 2-hydroxyglutarate (2-HG), leading to the accumulation of 2-HG and potentially impairing cell differentiation, as well as promoting the proliferation of tumor cells.
Ivosidenib, an oral IDH1 inhibitor, is currently recommended by the National Comprehensive Cancer Network (NCCN) as a second-line therapy for advanced, unresectable, and metastatic BTC harboring IDH1 mutations[21]. The approval of ivosidenib by the Food and Drug Administration (FDA) was based on the compelling results of the phase III CLARIDHY study[22]. This trial showed a statistically significant improvement in PFS for patients randomized to ivosidenib, with a HR of 0.37 (95%CI: 0.25-0.54;
Approved targeted therapies for BTC
Target | Drug | Trial name | Patient number (n) | ORR | mPFS (months) | mOS (months) | Grade ≥ 3 TRAE |
IDH | Ivosidenib | ClarIDHy | 187 | - | 2.7 | 10.3 | 53% |
FGFR | Pemigatinib | FIGHT-202 | 146 (107 FGFR R/F + 20 FGFR-O + 18 FGFR-N + 1 unassigned) | 35.5% (in FGFR R/F) | 6.9 (in FGFR R/F) | 21.1 (in FGFR R/F) | 64% |
Futibatinib | FOENIX-CCA2 | 103 | 41.7% | 8.9 | 20.0 | 73.1% | |
BRAF/MEK | Dabrafenib/ Trametinib | ROAR | 43 | 51% | 9 | 14 | 53% |
HER2 | Trastuzumab/ Pertuzumab* | MyPathway | 39 | 23 (0% in iCCA) | 4 (2.6 in iCCA) | 10.9 (3.9 in iCCA) | 46% |
TRK | Larotrectinib | LOXO-TRK-14001, SCOUT, NAVIGATE | 244 | 69% | 29.4 | NR | 20% |
Entrectinib | ALKA, STARTRK-1, STARTRK-2 | 150 | 61.3% | 13.8 | 37.1 | - | |
RET | Pralsetinib* | ARROW | 23 including 3 BTC | 57% | 7.4 | 13.6 | 69% |
Selpercatinib | LIBRETTO-001 | 41 including 2 BTC | 43.9% | 13.2 | 18.0 | 38% |
Several ongoing early-phase trials are assessing other IDH inhibitors in IDH mutant tumors including BTC such as LY3410738 (NCT04521686)[23], HMPL-306 (NCT04762602)[24], and IDH305 (NCT02381886)[25]. Additionally, there is an ongoing study (NCT04088188) evaluating the addition of ivosidenib with gemcitabine and cisplatin as a treatment for advanced BTC patients[26]. Although it is a phase I study, it holds the potential to provide valuable insights into the efficacy of combining targeted therapy and chemotherapy in the management of advanced BTC. A summary of ongoing targeted therapy trials with data is provided in [Table 3].
Ongoing targeted therapy trials in BTC
Target | Drug/Name of trial | Phase | Trial ID | Outcome | Note |
IDH | LY3410738 | I | NCT04521686 | LY3410738 is a dual IDH1/2 inhibitor | |
HMPL-306 | I | NCT04762602 | HMPL-306 is a dual IDH1/2 inhibitor | ||
IDH305 | I | NCT02381886 | IDH1R132-mutant tumors are eligible | ||
ivosidenib + gemcitabine + cisplatin | I | NCT04088188 | |||
FGFR | Futibatinib/ FOENIX-CCA3 | III | NCT04093362 | Futibatinib versus Gemcitabine + Cisplatin as a first-line in advanced BTC with FGFR2 gene rearrangement | |
Derazabtinib/ FIDES-01 | II | NCT03230318 | In FGFR2 fusion group: ORR 21.4%, mPFS 8.0 months, mOS 17.2 months[27] | Derazabtinib is a pan-selective FGFR inhibitor | |
RLY-4008/ ReFocus | I/II | NCT04526106 | ORR 88.2%[28] | RLY-4008 is a highly selective FGFR2 inhibitor | |
Pemigatinib/ FIGHT-302 | III | NCT03656536 | Pemigatinib versus Gemcitabine/Cisplatin as a frontline in advanced BTC with FGFR2 rearrangement | ||
KIN-3248 | I/Ib | NCT05242822 | KIN-3248 is a selective small molecule pan-FGFR inhibitor | ||
Bemarituzumab | Ib/II | NCT05325866 | Bemarituzumab is a humanized IgG1 monoclonal antibody against FGFR2b | ||
BRAF | ABM-1310 | I | NCT05501912 | ABM-1310 is a small molecule BRAF inhibitor | |
NCT04190628 | |||||
BGB-3245 | Ia/Ib | NCT04249843 | BGB-3245 is a small molecule inhibitor of RAF monomer and dimer | ||
HER2 | Trastuzumab deruxtecan/ HERB | II | JMA-IIA00423 | ORR 36.4%, DCR 81.8%, mPFS 4.4 months, mOS 7.1 months. Grade ≥ 3 TRAEs in 81.3% of patients[29] | |
Zanidatamab/ HERIZON-BTC-01 | IIb | NCT04466891 | ORR 41.3%, DCR 68.8%, mPFS 5.5 months, OS at 9 months of 69.9%[30] | Zanidatamab is a HER2-targeted bispecific antibody | |
DB-1303 | I/IIa | NCT05150691 | DB-1303 is an ADC consisting of a humanized anti-HER2 IgG1 monoclonal antibody | ||
Tucatinib + trastuzumab and oxaliplatin-based therapy or pembrolizumab-containing combinations | Ib/II | NCT04430738 | |||
CT-0508 | I | NCT04660929 | CT-0508 is a CAR-macrophage |
The promising data on IDH inhibitors in BTC hold potential, but challenges, especially regarding acquired resistance, must be addressed. Studies are investigating the mechanisms of secondary resistance to IDH inhibitors. However, the molecular underpinnings of this resistance are not yet fully elucidated. Several studies have found mutant IDH isoform switching as a potential mechanism of acquired resistance to IDH-targeted therapy[31,32]. Nonetheless, additional research is necessary to gain a comprehensive grasp of these molecular processes.
Fibroblast growth factor receptor inhibitors
FGFR is a cell surface receptor that transmits signals from fibroblast growth factors, playing an essential role in regulating cell proliferation, tissue maturation, and cellular differentiation[33]. Continuous activation of FGFRs can lead to the proliferation of cancer cells. Activation of FGFRs can occur via different mechanisms, including gene amplification, which results in the overexpression of receptors, activating mutations, or translocations that create activating gene fusions[34]. Among all FGFR alterations, FGFR2 fusions exhibit the highest frequency in BTC, being detected in approximately 10%-15% of patients with iCCA, while being comparatively rare in other subtypes[35,36]. Several pan-FGFR inhibitors and selective FGFR inhibitors have been undergoing investigation. The data obtained from registrational studies provide compelling evidence that FGFR2 fusions, as the most prevalent alterations in BTC, significantly contribute to the therapeutic efficacy observed in this disease[37,38].
Pemigatinib and futibatinib are two FGFR inhibitors currently available for patients with pretreated BTC who have FGFR2 fusions or rearrangements[39]. In April 2020, the FDA granted accelerated approval to an oral inhibitor of FGFR1-3, pemigatinib, as a therapy option for previously treated advanced BTC patients who tested positive for FGFR2 fusion or FGFR2 rearrangement, based on the findings of the phase II FLIGHT-202 study[40,41]. The final analysis of the study, with a median follow-up duration of 45.4 months, showed a disease control rate (DCR) of 82.4% (95%CI: 73.9%-89.1%) and a median OS of 17.5 months (95%CI: 14.4-22.9 months)[42]. Based on the promising data mentioned earlier, a phase III randomized study, FIGHT-302 (NCT03656536), is currently underway to evaluate pemigatinib as a frontline treatment for advanced BTC patients, comparing it with the combination of gemcitabine and cisplatin[43].
Futibatinib, an oral agent that inhibits FGFR 1-4, has received accelerated approval from the FDA in September 2022[44]. This decision was based on the demonstrated efficacy in patients with previously treated advanced or metastatic iCCA with FGFR fusions or rearrangements, as shown in the data from the phase II FOENIX-CCA2 trial[45]. An updated analysis data, with a median follow-up of 25 months, revealed an objective response rate (ORR) of 41.7% with a DCR of 82.5%[46]. The median duration of response (DOR) was 9.5 months, while the median PFS was 8.9 months. Furthermore, the mature median OS was recorded as 20.0 months. Similarly to pemigatinib, a phase III clinical trial (FOENIX-CCA3, NCT04093362) is currently underway. This trial is evaluating futibatinib as a frontline option for locally advanced, metastatic, or recurrent unresectable iCCA with FGFR2 rearrangements. The trial aims to compare the efficacy of futibatinib with the doublet of gemcitabine and cisplatin.
Both studies evaluating pemigatinib and futibatinib showed a similar side effect profile. Hyperphosphatemia, alopecia, and diarrhea were the most frequent TRAEs noted with both drugs. In the case of pemigatinib, these side effects were mostly in grade 1 or 2. Among grade 3 or more severe adverse events, hypophosphatemia was the most frequently reported, occurring in 14.3% of cases[42]. Similarly, for futibatinib, the most common grade 3 TRAE was hyperphosphatemia, seen in 30% of participants[45]. Additionally, other notable grade 3 adverse events included increased aspartate aminotransferase levels (7%), stomatitis (6%), and fatigue (6%).
Several ongoing trials hold the potential to provide promising data in the near future. One of these is the phase II FIDES-01 study, investigating derazantinib, a pan-selective FGFR inhibitor, in patients with pretreated iCCA harboring FGFR2 fusion (FGFR2F) or FGFR mutation/amplification (FGFR2M/A). Data from the 2022 ESMO Congress showed a higher ORR of 21.4% (95%CI: 13.9%-30.5%) in FGFR2F compared to 6.5% in FGFR2M/A cohort (95%CI: 0.8%-21.4%)[29]. The median OS was documented as 17.2 months (95%CI: 12.5%-22.4%) and the median PFS reached 8.0 months (95% CI: 5.5%-8.3%) in FGFR2F patients.
In the ongoing ReFocus trial (NCT04526106), RLY-4008, a highly selective FGFR2 inhibitor, is being evaluated in advanced solid tumors, including FGFR inhibitor-naïve BTC. Preliminary data from the 2022 ESMO Congress showed a promising ORR of 88.2% (95%CI: 63.6%-98.5%) in 17 patients who received the recommended phase 2 doses[30]. Notably, one patient achieved a near-complete response and underwent curative tumor resection. The encouraging findings underscore the significance of further monitoring the ongoing development of RLY-4008 as a potential treatment option for advanced stages and potentially in the neoadjuvant setting.
The encouraging data on FGFR inhibitors in BTC holds promise, but there are significant challenges to overcome, particularly concerning acquired resistance to these inhibitors. Numerous preclinical investigations have been conducted to identify the underlying mechanisms of resistance, including bypass signaling, epithelial-mesenchymal transition (EMT), and the development of secondary FGFR mutations, commonly referred to as gatekeeper mutations[47]. However, it is vital to acknowledge that the majority of research in this area has primarily centered on urothelial, lung, and gastric cancer cell lines, potentially not encompassing the complete range of resistance mechanisms observed in BTC. Moreover, in vitro cell models may not entirely capture the heterogeneity and complexity of human disease, adding another layer of consideration in interpreting the findings and applying them to BTC treatment strategies.
BRAF inhibitors
The mitogen-activated protein kinase (MAPK) signaling pathway, which includes the RAS/RAF/MEK/ERK pathway, plays an essential role in cellular proliferation and survival[48]. BRAF, an oncogene, activates the RAS/RAF/MEK pathway. BRAF mutations, particularly the V600E mutation, are commonly observed in various solid tumors, including colorectal malignancy, non-small cell lung cancer (NSCLC), and melanoma[49,50]. The BRAF V600E mutation activates BRAF, resulting in tumor growth and metastasis[51]. BRAF activating mutations are rare in BTC, comprising approximately 5.5% of cases, with 1.5% of BRAF V600E mutation in iCCA and 0.5% of BRAF V600E in GBC[12].
Combining the RAF inhibitor, dabrafenib, with the MEK inhibitor, trametinib, has shown encouraging results in the treatment of BRAF-mutated BTC. The phase II ROAR trial investigated the effectiveness of combining dabrafenib and trametinib in patients with advanced solid tumors harboring the BRAFV600E mutation who had experienced the progression of disease after prior treatment[52]. The trial showed a significant ORR of 51%, with a mean OS of 14 months and a mean PFS of 9 months. The dabrafenib and trametinib combination regimen demonstrated manageable side effects. In light of these results, the FDA approved dabrafenib plus trametinib as a subsequent line of therapy for BTC with BRAFV600E mutations[53].
Ongoing phase 1 trials are exploring the response to selective BRAF inhibitors, such as ABM-1310 (NCT05501912, NCT04190628) and BGB-3245 (NCT04249843), in patients with BRAFV600-mutated solid tumors, including BTCs. Additionally, more studies are needed to evaluate the effectiveness of targeted therapies in patients with concurrent TP53 and BRAFV600E mutations, as initial findings suggest a more aggressive disease and reduced clinical benefits[54].
Similar to other targeted therapies, the challenge of overcoming resistance to BRAF inhibitors persists. As evidenced by PFS data, a significant proportion of patients initially responsive to BRAF inhibitors eventually face resistance, necessitating adjustments in treatment strategies. While several potential mechanisms have been proposed, the majority of investigations have revolved around melanoma and a limited range of lung cancer cohorts. Nevertheless, a comprehensive analysis of these findings offers relevant insights that can be extrapolated to BTC patient populations. Notably, one of the potential mechanisms involves the reactivation of the MAPK pathway[55-57], often occurring due to alterations affecting the drug target itself, such as BRAF copy number gains and MEK2 mutations[58-60].
As discussed earlier, combination treatment involving BRAF inhibitors and MEK inhibitors has shown promising results. However, despite this encouraging observation, the development of acquired resistance to combination therapy remains an inevitable challenge. A study conducted within a melanoma cohort has highlighted that the mammalian target of rapamycin (mTOR) activation could potentially contribute to the acquired resistance seen with combined BRAF and MEK inhibition[61]. The mTOR kinase plays an important role in cellular proliferation, and aberrant mTOR activation has been noted across various cancer types[62,63]. Given that both the MAPK/ERK and PI3K/AKT signaling pathways converge into the mTOR complex 1, this pathway could hold significance for the effectiveness of targeted therapy in patients with BRAF-mutant melanoma[61]. This potentially implies that the inclusion of an mTOR inhibitor might effectively restore sensitivity to BRAF or MEK inhibitors, prompting further exploration for potential therapeutic interventions.
Human epidermal growth factor receptor 2 inhibitors
HER2, encoded by the ERBB2 gene, is a receptor tyrosine kinase involved in crucial oncogenesis signaling pathways. Its dysregulation is well-documented in cancer development[64]. Besides its well-known significance in breast cancer, emerging evidence suggests its involvement in BTC[65]. HER2 expression shows ethnic variations, with a higher prevalence in Asian patients (28.4%) compared to Western patients
Pertuzumab and trastuzumab, two monoclonal antibodies targeting HER2, have shown promise in treating HER2-positive malignancies[68]. In a phase II MyPathway study (NCT02091141) involving previously treated HER2-amplified and overexpressed metastatic BTC patients, the pertuzumab and trastuzumab combination demonstrated an ORR of 23% (95%CI: 11%-39%), a median PFS of 4.0 months (95%CI: 1.8-5.7 months), and a median DOR of 10.8 months (95%CI: 0.7-25.4 months)[69]. Adverse events of grade 3 or higher were documented in 46% of patients, with alanine aminotransferase (ALT) and aspartate aminotransferase (AST) increases being the most common. Although the dual anti-HER2 therapy for HER2-positive BTC has not received FDA approval, it is included as an option in the NCCN guidelines for patients who have undergone prior treatment[21]. To further confirm and establish the efficacy of the HER2-targeted treatment in subsequent lines of therapy for BTC, future randomized controlled trials are essential.
Trastuzumab deruxtecan (T-DXd) has demonstrated encouraging anti-cancer efficacy in advanced solid tumors that are HER2-positive, including BTC[70]. A phase I study involving advanced HER2 mutant solid tumors, excluding breast and gastric cancers, showed an ORR of 28.3%, and the median PFS reached 7.2 months (95%CI: 4.8-11.1 months). The HERB phase II study, which focused on BTCs, revealed an ORR of 36.4% (95%CI: 19.6%-56.1%) in patients with HER2-positive BTCs, along with a DCR of 81.8%
Zanidatamab, a HER2-targeted bispecific antibody, underwent evaluation in the phase IIb HERIZON-BTC-01 study (NCT04466891) in patients with HER2-amplified, locally advanced, unresectable, or metastatic BTC. Among the 87 patients, 41.3% of participants had an objective response, with a DCR of 68.8%
Despite remarkable advances in HER2-targeted therapy, resistance continues to pose challenges in HER2-positive BTCs. While resistance studies are primarily focused on breast and gastrointestinal cancers, they provide valuable insights into potential resistance mechanisms in BTC. Notably, the intrapatient and intertumor heterogeneity of HER2 expression is considered a critical factor contributing to primary resistance[71,72]. Furthermore, HER2 positivity in BTC often coincides with other oncogenic drivers such as FGFR, MET, and KRAS alterations, known to impart resistance to anti-HER2 therapy in in vitro studies[73].
Encouragingly, a small case series demonstrated the successful reversal of trastuzumab resistance by combining HER2 blockade with inhibition of the secondary driver mutation[74]. Additionally, in studies involving gastric cancer patients, loss of HER2 has been linked to potential acquired resistance to anti-HER2 therapy[75,76]. Notably, the SUMMIT study evaluated an irreversible pan-HER tyrosine kinase inhibitor, neratinib, in HER2-mutant advanced BTC, showing antitumor activity but not meeting the primary endpoint; in one GBC patient, loss of HER2 amplification and reduction in VAF of the original HER2 mutation were demonstrated through biopsy and NGS[77]. Combinatorial approaches, continuous monitoring of HER2 alterations, and adaptive treatment regimens may offer potential solutions to improve clinical outcomes and enhance the efficacy of HER2-targeted therapy in the management of BTCs.
Tropomyosin receptor kinase inhibitors
The neurotrophic tropomyosin kinase receptors (NTRK or TRK) family is a group of transmembrane tyrosine kinases that hold significance in the development of neurons. These receptors are encoded by the genes NTRK1, NTRK2, and NTRK3. In 1986, the first identification of somatic fusions involving the NTRK genes was reported in a colorectal cancer patient. Subsequently, it was uncovered that mutations in NTRK genes, most commonly NTRK fusions, are associated with oncogenesis in various tumor types. The NTRK mutations are more common in patients with lung cancers and soft tissue tumors[78]. The estimated prevalence of NTRK fusions in BTC is 0.75%[15].
To date, the FDA has approved two first-generation NTRK inhibitors as first-line and subsequent line of therapy in patients with NTRK fusion-positive BTC: larotrectinib[79] and entrectinib[80]. In November 2018, the FDA granted accelerated approval to Larotrectinib based on results from three multicenter clinical trials: LOXO-TRK-14001, SCOUT, and NAVIGATE. A pooled analysis of the three studies showed an ORR of 69% (95%CI: 63-75), with more than one-fourth of the cases (26%) achieving a complete response (CR)[81]. The response has shown considerable durability, with a median DOR of 32.9 months (95%CI: 27.3-41.7). The median PFS reached 29.4 months (95%CI: 19.3-34.3). The median was not reached at a median follow-up of 32.2 months. The TRAEs were primarily in grade 1 or 2, and 20% of patients had grade 3 or 4 adverse events, leading to treatment discontinuation in five cases.
Entrectinib was approved by the FDA in the subsequent year for adults and pediatric patients who have solid tumors with NTRK gene fusion based on favoring data from three multicenter, single-arm clinical trials: ALKA, STARTRK-1 (NCT02097810) and STARTRK-2 (NCT02568267)[82]. An updated pooled analysis data of the three phase 1-2 trials from the 2022 ASCO annual meeting has demonstrated meaningful clinical response and good tolerability[83]. The ORR was 61.3% (95%CI: 53.1%-69.2%), with more than one-fourth of those who had responses achieving a CR. The median DOR reached 20.0 months (95%CI: 13.2-31.1 months) and the PFS reached 13.8 months (95%CI: 10.1-20.0 months). At a median survival follow-up of 30.6 months, the median OS reached 37.1 months (95%CI: 27.2-NE). Moreover, the TRAEs were mainly grade 1-2: dysgeusia (36.6%), diarrhea (29.8%), and weight gain (28.5%). A total of 7.2% of patients needed to discontinue the treatment due to adverse events.
While a significant portion of patients with NTRK fusion-positive cancers achieve enduring disease control, the emergence of resistance to TRK inhibition remains a substantial challenge[84]. This emphasizes the critical need to develop second-generation TRK inhibitors and/or explore strategies to counter this resistance mechanism. A recent study examining post-progression tumor tissue through NGS from patients treated with first-generation TRK inhibitors found that the majority displayed on-target resistance (83%) as opposed to off-target resistance (11%) or an unidentifiable mechanism (6%)[85]. Among patients exhibiting on-target resistance, mutations primarily affected the solvent front (87%) and, to a lesser extent, the gatekeeper region (13%). The sequential use of next-generation therapy appears to impact the pattern of mutation occurrence and development. At present, several next-generation TRK inhibitors, such as selitrectinib, repotrectinib, and taletrectinib, are undergoing evaluation in clinical trials due to their promising preclinical activity[86]. These innovative agents hold considerable potential in addressing the resistance challenge that may arise with the use of first-generation TRK inhibitors.
RET inhibitors
For over three decades following the identification of the RET gene that encodes the receptor tyrosine kinase, mutations in the RET gene have been identified as actionable drivers of oncogenesis[87]. Specific RET fusion proteins can initiate tumorigenesis and progression of cancer by stimulating subsequent signaling cascades, ultimately resulting in unregulated cell growth. While RET gene fusions are relatively rare in BTC, they are identified in around 1% to 2% of other types of cancer, such as NSCLC and thyroid cancers, indicating the promise of targeting RET kinase in therapeutic interventions[88]. Activating aberrations in RET, including mutations, fusions/rearrangements, or amplifications, are found in about 1.8% of all tumors, with a favorable response observed in many cases to RET-directed therapy[89]. Despite the overall rarity of RET aberrations, their significance in driving oncogenesis highlights the need for further research and understanding in order to explore potential therapeutic interventions.
Pralsetinib, a selective RET receptor tyrosine kinase inhibitor, has shown encouraging results in the phase 1/2 ARROW trial, where it was evaluated in patients with solid tumors carrying RET fusions, including BTC[90]. The study showed an ORR of 57% (95%CI: 35%-77%), with a DOR of 11.7 months (95%CI: 5.5-19.0 months). The median PFS reached 7.4 months (95%CI: 5.1-13.6 months) and the median OS reached 13.6 months (95%CI: 7.5-NE) with a median follow-up of 23.5 months. Among the three BTC patients, two participants had objective responses. In the safety analysis of 29 eligible patients, 20 patients (69%) experienced grade ≥ 3 TRAEs, with the most frequent any-grade TRAEs being abnormal liver function tests. Based on these findings, pralsetinib was approved by the FDA for advanced or metastatic RET-fusion-positive lung and thyroid cancers[91,92]. While pralsetinib has not received FDA approval as a tumor-agnostic drug, it is listed as a recommended option in the NCCN guidelines for both first-line and subsequent-line treatment of RET-positive BTC[21].
Selpercatinib, a highly selective RET kinase inhibitor, was approved by the FDA for the treatment of any RET-mutated solid tumors following promising data from the LIBRETTO-001 trial, a phase 1/2 basket trial assessing its efficacy in solid tumors with RET fusion, regardless of their primary location[93,94]. Among 41 efficacy-evaluable patients, the ORR reached 43.9% (95%CI: 28.5-60.36), with a DOR of 24.5 months (95%CI: 9.2-NE), including two BTC patients, one of whom had a response. The PFS was 13.2 months (95%CI: 7.4-26.6) and the OS reached 18.0 months (95%CI: 10.7-NE). 38% of patients had grade ≥ 3 TRAEs, with hypertension and abnormal liver function tests being frequently observed as grade 3 TRAEs. Selpercatinib is currently recommended in the NCCN guidelines for both progressive and first-line treatment of RET-positive BTC[21]. Furthermore, its CNS activity highlights its potential in treating locally advanced or metastatic solid tumors harboring RET fusions.
RET fusions exhibit diverse patterns, and the impact of fusion partners and breakpoints on drug response is not fully understood[95]. Despite an initial positive response, acquired resistance to RET inhibition treatment inevitably emerges[96]. While data on acquired resistance to RET inhibitors is growing, a comprehensive understanding is not yet matured. Recent data in lung and thyroid cancer patients suggest that primary and acquired resistance mechanisms converge on MAPK pathway activation[97]. Therefore, employing a sequential approach to RET-targeted therapy could require a combination of treatments involving inhibitors that target distinct MAPK pathways. Furthermore, it appears necessary to reevaluate the characterization of tumors treated with RET inhibitors when they show progression under RET inhibitor therapy.
IMMUNOTHERAPIES FOR BILIARY TRACT CANCER
High tumor mutational burden
Programmed cell death ligand-1(PD-L1) is an immune checkpoint ligand that allows cancer cells to evade immune recognition[98]. Immunotherapy targeting PD-L1 or its receptor, programmed cell death 1 (PD-1), has shown promising results in various cancers. Hypermutated tumors with a high tumor mutational burden (TMB) are particularly responsive to immune checkpoint inhibitors[99,100]. TMB is the number of somatic gene mutations present in a tumor and it serves as a biomarker for predicting the response to immunotherapy[101]. TMB-H, with a threshold of ≥ 10 mutations per megabase, indicates a high probability of neoantigen formation and has been associated with improved outcomes in several cancer types. Approximately 4% of all subtypes of BTCs are estimated to have TMB-H[19].
Pembrolizumab, an immune checkpoint inhibitor targeting PD-1 receptors, was approved by the FDA for the treatment of TMB-H tumors[102]. The KEYNOTE-158 trial assessed pembrolizumab in TMB-H tumors across various sites[103]. The study revealed a 29% ORR (95%CI: 21%-39%). Additionally, 57% of participants achieved a DOR lasting 12 months or longer, and 50% attained a DOR of 24 months or longer. Although there were 63 BTC patients in the KEYNOTE-158 trial, none of them had high TMB. However, two out of the 63 patients had objective responses.
Recent data from the phase II CheckMate-848 study (NCT03668119) demonstrated promising results using nivolumab and ipilimumab in patients with previously treated advanced or metastatic TMB-H solid tumors[104]. The ORR was 35.5% (95%CI: 24.1%-47.8%) in the group with tissue TMB-H and 22.5% (95%CI: 13.9%-33.2%) in the group with blood TMB-H. Based on the above findings, the current NCCN guidelines list nivolumab and ipilimumab combination therapy as frontline and subsequent-line treatment for TMB-H BTCs, and pembrolizumab is listed as a subsequent-line treatment for TMB-H BTCs[21].
High microsatellite instability and mismatch repair deficient
Tumors with mismatch repair deficiency (dMMR) or high microsatellite instability (MSI-H) levels show increased neoantigen production and CD8+ T cell infiltration[105]. MSI-H/dMMR leads to difficulties in repairing DNA replication errors, causing mutations. The MSI-H/dMMR is identified in about 2.06% of iCCA patients[17].
Pembrolizumab has received full FDA approval for the treatment of adult and pediatric patients with unresectable or metastatic MSI-H or dMMR solid tumors, who have progressed after prior treatment and have limited alternative options[106]. The approval is based on data from multiple trials, including KEYNOTE-158 (NCT02628067), KEYNOTE-164 (NCT02460198), and KEYNOTE-051 (NCT02332668), involving 504 patients with over 30 types of tumors. An integrated analysis of these studies revealed that, at a median follow-up of 20.1 months, pembrolizumab achieved an ORR of 33.3% (95%CI: 29.2%-37.6%), comprising a CR rate of 10.3% and a partial response rate of 23.0%. According to the NCCN guidelines, pembrolizumab is currently listed as both a first-line and subsequent-line treatment option for MSI-H/dMMR tumors[21].
Dostarlimab, another anti-PD-1 monoclonal antibody, was assessed in the phase I GARNET trial. This study included 106 patients who had advanced solid tumors, and two of them had MSI-H/dMMR BTCs[107]. The trial showed that an ORR at 38.7% (95%CI: 29.4%-48.6%) in patients with dMMR and CR was around 7.5%. A median DOR was not reached with a median follow-up of 12.4 months. About 8.3% of patients experienced at least one grade 3 or higher adverse event, with the most common being an elevated lipase level. Based on this, the FDA approved dostarlimab for recurrent or advanced solid tumors with MSI-H/dMMR, which have progressed after previous lines of therapy and do not qualify for suitable alternative treatment options[108].
Adoptive immunotherapy
While immune checkpoint inhibitors have made notable progress, their effectiveness in treating BTC remains unsatisfactory. Another promising approach in the field of BTC immunotherapy is Chimeric Antigen Receptor (CAR) T-cell therapy, which is currently being evaluated. CAR T-cell therapy has demonstrated exceptional efficacy in the treatment of hematologic cancers, having received regulatory approval for conditions such as B-cell lymphoma. However, expanding the use of CAR T-cell treatment to solid tumors, such as BTC, presents unique challenges due to these tumors' ability to exclude T cells from the tumor microenvironment and avoid immune system recognition.
Researchers have been actively exploring the potential of introducing highly potent cancer-specific T cells into solid tumors as an innovative strategy. Encouraging findings have been observed, particularly with fourth-generation anti-CD133 CAR T-cells, which have demonstrated significant effectiveness against BTC cells expressing CD133[109]. Additionally, the combination of CAR T therapies targeting both EGFR and CD133 in a cocktail immunotherapy approach has produced promising outcomes in advanced BTC patients[110]. Furthermore, a phase I study (NCT01869166) involving EGFR-specific CAR T cells for EGFR-mutated advanced BTC has shown favorable results, including a 5.8% CR rate and stable disease in 58.8% of patients[111].
Moreover, at the 2023 ASCO meeting, results from a phase I trial using gavocabtagene autoleucel, an autologous genetically engineered anti-mesothelin T cell receptor fusion construct cell therapy, were presented[112]. This trial focused on patients with refractory mesothelioma and other mesothelin-expressing solid tumors, including BTC. The results were promising, with an ORR of 21% and a DCR of 77% among the 32 patients who participated in the study, including one patient with BTC. Furthermore, the 6-month OS rate reached 70.2%. These findings suggest a potential avenue for effective treatment in patients with mesothelioma patients and other mesothelin-expressing solid tumors, offering hope in the fight against these challenging malignancies. Nevertheless, concerns about the toxicity of CAR T-cell therapy in BTC persist, mainly due to potential toxicity and endothelial damage, emphasizing the imperative for further investigation and the conduct of clinical trials.
In conclusion, while CAR T-cell therapy has significantly advanced the treatment of hematologic malignancies, its application for solid tumors, including BTC, brings forth a landscape filled with both promise and hurdles. Research involving CAR T cells targeting specific antigens such as CD133 and EGFR has demonstrated effectiveness in both preclinical and clinical contexts, instilling hope for patients with conditions like BTC. Nonetheless, persisting safety concerns and the imperative for more extensive research underscore the continuous endeavors to enhance the potential of CAR T-cell therapies for solid tumors, with the ultimate goal of improving their efficacy while mitigating potential adverse effects.
CHALLENGES AND POTENTIALS OF TARGETED THERAPIES
Sequencing of cancer genomics
The evolution of targeted therapy is closely intertwined with the advancements in DNA sequencing technology used in cancer cells. Cancer, being a genomic disease, is often influenced by both somatic and germline mutations, highlighting the crucial need to comprehend cancer's genomic sequencing in order to uncover its origins and develop personalized treatment approaches. Recent progress and cost reductions in NGS have greatly accelerated the transformation of cancer genomic data into practical clinical applications. It allows simultaneous analysis of hundreds or thousands of genes, empowering the implementation of molecularly targeted therapy based on the insights gained from the results.
NGS technology has enabled the identification of genomic profiles for individual cancer cells, paving the way for personalized treatment approaches. However, the diversity of tumor genomic sub-categories identified by NGS makes it exceedingly difficult to conduct randomized controlled trials with an adequate number of patients for each specific sub-category[113]. Furthermore, the intratumoral environment consists of diverse and heterogeneous cells, resulting in variations in genomic profiles and mutation data among these cells. As a consequence, there are concerns about the feasibility of relying solely on sequencing results to determine the most suitable targeted therapy[114].
Resistance and potential overcome strategies
The iCCA develops resistance to treatments, which is closely associated with cancer-related mortality, although the precise mechanisms underlying this resistance are not fully understood. During cancer treatment, adaptive changes lead to acquired resistance, involving mechanisms such as genetic alterations, changes in the tumor's microenvironment, metabolic reprogramming, and loss of the treatment target[115,116].
One of the investigational methods to elucidate the development of acquired resistance in cancer, as observed in targeted therapy, involves analyzing the molecular differences between tumor samples before and after acquiring resistance[117]. By comparing the resistant tumor sample with the pre-treatment tumor sample at the molecular level, we can identify whether the resistance is due to changes in previously actionable mutations or the emergence of new mutations. This comprehensive analysis can potentially provide insights into the underlying causes of resistance and guide the modification of treatment strategies accordingly.
Furthermore, to overcome acquired resistance driven by tumor genetic alterations, exploring the next generation of inhibitors and researching strategies for combination treatments appears to be essential. By focusing on these areas of investigation, we can aim to develop more effective approaches to combat acquired resistance and enhance the overall efficacy of cancer therapies.
Determining the sequence of treatment
We have thoroughly reviewed various systemic treatment options for advanced iCCA, along with the foundational research data. While we are aware of the several options available, the next consideration and decision-making process involve the prioritization and sequence of these treatment modalities. As previously mentioned, any systemic treatment may initially demonstrate a favorable response but ultimately develop acquired resistance due to various factors, necessitating a change in treatment.
Given the relatively recent use of targeted therapies and the rarity of actionable mutations among the iCCA patient population, conducting head-to-head comparing trials between targeted therapy and chemotherapy and/or immunotherapy is challenging. Consequently, selecting treatment strategies tailored to individual patient situations is essential. Elements shaping these individualized decisions encompass factors such as the extent of tumor burden, the urgency of treatment initiation takes into account the time required for sequencing the tumor genome, the patient's medical history, drug accessibility, financial aspects, anticipated toxicity, patient performance status, and anticipated treatment response.
In many cases, combining chemotherapy with immunotherapy would be a preferable first-line treatment while awaiting tumor genomic information. However, as the tumor progresses, established guidelines for selecting second-line therapy remain absent due to limited supportive data. Tailored to the unique context of individual patients, second-line treatment might encompass targeted therapy, immunotherapy, or FOLFOX, with reference to the ABC-06 trial findings, alongside the consideration of other cytotoxic chemotherapy treatments. Although direct comparative prospective study data could be lacking, using targeted therapy upfront and reserving FOLFOX could be a reasonable approach if the patient has any indication for targeted therapy. Targeted therapies are generally perceived as more tolerable and can exhibit considerable effectiveness, particularly when administered early, potentially yielding enhanced patient outcomes. The investigation into the sequencing of treatment strategies demands further exploration.
Potential role as neoadjuvant and adjuvant therapy
In patients with iCCA, complete resection is regarded as the sole definitive curative option, although its feasibility is limited for many due to the advanced disease at diagnosis. While not conclusively established, based on current data, hepatic resection with negative margins is considered the preferred surgical goal[118]. The available research data on neoadjuvant and adjuvant chemotherapy in resectable iCCA presents challenges in drawing definitive conclusions. A study result by Buettner et al. showed similar OS and PFS between patients with perioperative chemotherapy and those without, although this needs to be cautiously interpreted due to low neoadjuvant treatment patient numbers and possible selection bias effect[119]. Conversely, more recent research indicates that neoadjuvant therapy, particularly in stage II-III disease, may lead to enhanced OS[120-122]. Moreover, the optimal adjuvant treatment strategy for resected iCCA remains uncertain due to limited clinical trial data supporting a standard regimen.
The efficacy of targeted therapy as neoadjuvant or adjuvant treatment for resectable disease remains an unexplored area of research. The challenges of limited early-stage detections and infrequent actionable mutations hinder the conduct of studies in this domain. Moreover, the effects of targeted therapy on tumor downstaging, survival benefits, recurrence-free survival, and potential adverse events leading to unfavorable outcomes require further elucidation.
In an attempt to address this, a clinical trial (NCT05514912) is underway to evaluate chemo-targeted therapy for resectable intrahepatic cholangiocarcinoma using infigratinib in combination with chemotherapy in FGFR2 fusion-positive iCCA[123]. The study aims to provide valuable insights into the treatment efficacy for this specific patient subset. To our knowledge, there are currently no ongoing clinical trials investigating the efficacy of targeted therapy or combination treatments with targeted therapy in an adjuvant setting. Additional research is needed to address these uncertainties and shed light on potential treatment strategies.
CONCLUSION
In summary, iCCA remains a formidable challenge with historically poor outcomes. However, recent advancements in targeted therapies and immunotherapy offer hope for improved treatment strategies and better patient outcomes.
Targeted therapies, such as FGFR inhibitors, IDH inhibitors, and HER2 inhibitors, are demonstrating promising results in iCCA treatment by specifically targeting genetic alterations. These therapies provide personalized treatments, offering the potential for more effective responses and increased survival rates among iCCA patients.
Immunotherapies, including PD-L1 inhibitors and CAR T-cell therapy, have garnered significant attention as emerging treatment modalities in the context of BTC. Numerous ongoing research endeavors are focused on these approaches. Immune checkpoint inhibitors have gained FDA approvals for iCCA with TMB-H and MSI-H/dMMR, expanding the therapeutic options available for advanced or metastatic disease.
Despite these breakthroughs, challenges such as conducting large trials, overcoming acquired resistance, and optimizing treatment sequencing remain. Addressing these areas requires ongoing research and investigation. In conclusion, while iCCA treatment remains challenging, recent progress in targeted therapies and immunotherapy brings optimism. By advancing scientific understanding and clinical practice, we can pave the way for better outcomes for iCCA patients.
DECLARATIONS
Acknowledgments
I would like to acknowledge Donghyun Gim for providing assistance with creating the figure.
Authors’ contributions
Conceptualization: Badri N
Writing-original draft preparation: Gim G
Writing-review and editing: Badri N
Supervision: Badri N
Read and agreed to the published version of the manuscript: Gim G, Badri N
Availability of data and materials
Not applicable.
Financial support and sponsorship
None.
Conflicts of interest
Both authors declared that there are no conflicts of interest.
Ethical approval and consent to participate
Not applicable.
Consent for publication
Not applicable.
Copyright
© The Author(s) 2023.
REFERENCES
1. Banales JM, Cardinale V, Carpino G, et al. Expert consensus document: Cholangiocarcinoma: current knowledge and future perspectives consensus statement from the European Network for the Study of Cholangiocarcinoma (ENS-CCA). Nat Rev Gastro Hepat 2016;13:261-80.
2. Nagorney DM, Donohue JH, Farnell MB, Schleck CD, Ilstrup DM. Outcomes after curative resections of cholangiocarcinoma. Arch Surg 1993;128:871-7; discussion 877-9.
3. Rizzo A, Brandi G. Neoadjuvant therapy for cholangiocarcinoma: a comprehensive literature review. Cancer Treat Res Commun 2021;27:100354.
4. Lamarca A, Palmer DH, Wasan HS, et al. on behalf of the Advanced Biliary Cancer (ABC) Working Group. ABC-06 | A randomised phase III, multi-centre, open-label study of active symptom control (ASC) alone or ASC with oxaliplatin / 5-FU chemotherapy (ASC+mFOLFOX) for patients (pts) with locally advanced / metastatic biliary tract cancers (ABC) previously-treated with cisplatin/gemcitabine (CisGem) chemotherapy. JCO 2019;37:4003.
5. Ramírez-Merino N, Aix SP, Cortés-Funes H. Chemotherapy for cholangiocarcinoma: An update. World J Gastrointest Oncol 2013;5:171-6.
6. Valle J, Wasan H, Palmer DH, et al. ABC-02 Trial Investigators. Cisplatin plus gemcitabine versus gemcitabine for biliary tract cancer. N Engl J Med 2010;362:1273-81.
7. Oh D, Ruth He A, Qin S, et al. Durvalumab plus gemcitabine and cisplatin in advanced biliary tract cancer. NEJM Evidence 2022:1.
8. Lowery MA, Ptashkin R, Jordan E, et al. Comprehensive molecular profiling of intrahepatic and extrahepatic cholangiocarcinomas: potential targets for intervention. Clin Cancer Res 2018;24:4154-61.
9. Abou-Alfa GK, Macarulla T, Javle MM, et al. Ivosidenib in IDH1-mutant, chemotherapy-refractory cholangiocarcinoma (ClarIDHy): a multicentre, randomised, double-blind, placebo-controlled, phase 3 study. Lancet Oncol 2020;21:796-807.
10. Montal R, Sia D, Montironi C, et al. Molecular classification and therapeutic targets in extrahepatic cholangiocarcinoma. J Hepatol 2020;73:315-27.
11. Ross JS, Wang K, Gay L, et al. New routes to targeted therapy of intrahepatic cholangiocarcinomas revealed by next-generation sequencing. Oncologist 2014;19:235-42.
12. Li W, Cui Y, Yin F, et al. BRAF mutation in Chinese biliary tract cancer patients. JCO 2020;38:e16678-e16678.
13. Mondaca S, Razavi P, Xu C, et al. Genomic characterization of ERBB2-driven biliary cancer and a case of response to ado-trastuzumab emtansine. JCO Precis Oncol 2019;3:PO.19.00223.
14. Thein KZ, Biter AB, Banks KC, et al. Identification of KRASG12C mutations in circulating tumor DNA in patients with cancer. JCO Precis Oncol 2022;6:e2100547.
16. Parimi V, Tolba K, Danziger N, et al. Genomic landscape of 891 RET fusions detected across diverse solid tumor types. NPJ Precis Oncol 2023;7:10.
17. Yu J, Zhang X, Huang Q, Tan S, Xiong X, Gou H. Rare DNA mismatch repair-related protein loss in patients with intrahepatic cholangiocarcinoma and combined hepatocellular-cholangiocarcinoma and their response to immunotherapy. Cancer Manag Res 2021;13:4283-90.
18. Silva VW, Askan G, Daniel TD, et al. Biliary carcinomas: pathology and the role of DNA mismatch repair deficiency. Chin Clin Oncol 2016;5:62.
19. Shao C, Li G, Huang L, et al. Prevalence of high tumor mutational burden and association with survival in patients with less common solid tumors. JAMA Netw Open 2020;3:e2025109.
20. Tian W, Zhang W, Wang Y, et al. Recent advances of IDH1 mutant inhibitor in cancer therapy. Front Pharmacol 2022;13:982424.
21. NCCN clinical practice guidelines in oncology (NCCN Guidelines®) biliary tract cancers, version 2.2023. Available from: https://www.nccn.org/professionals/physician_gls/pdf/btc.pdf [Last accessed on 25 Dec 2023].
22. FDA approves ivosidenib for advanced or metastatic cholangiocarcinoma. Available from: https://www.fda.gov/drugs/resources-information-approved-drugs/fda-approves-ivosidenib-advanced-or-metastatic-cholangiocarcinoma [Last accessed on 25 Dec 2023].
23. Study of LY3410738 administered to patients with advanced solid tumors with IDH1 or IDH2 mutations. Available from: https://classic.clinicaltrials.gov/ct2/show/NCT04521686 [Last accessed on 25 Dec 2023].
24. A study of HMPL-306 in advanced solid tumors with IDH mutations. Available from: https://classic.clinicaltrials.gov/ct2/show/NCT04762602 [Last accessed on 25 Dec 2023].
25. A study of IDH305 in patients with advanced malignancies that harbor IDH1R132 mutations. Available from: https://classic.clinicaltrials.gov/ct2/show/NCT02381886 [Last accessed on 25 Dec 2023].
26. Gemcitabine and cisplatin with ivosidenib or pemigatinib for the treatment of unresectable or metastatic cholangiocarcinoma. Available from: https://classic.clinicaltrials.gov/ct2/show/NCT04088188 [Last accessed on 25 Dec 2023].
27. Borad M, Javle M, Shaib W, et al. 59P Efficacy of derazantinib in intrahepatic cholangiocarcinoma (iCCA) patients with FGFR2 fusions, mutations or amplifications. Ann Oncol 2022;33:S567-8.
28. Hollebecque A, Borad M, Goyal L, et al. LBA12 Efficacy of RLY-4008, a highly selective FGFR2 inhibitor in patients (pts) with an FGFR2-fusion or rearrangement (f/r), FGFR inhibitor (FGFRi)-naïve cholangiocarcinoma (CCA): ReFocus trial. Ann Oncol 2022;33:S1381.
29. Ohba A, Morizane C, Kawamoto Y, et al. Trastuzumab deruxtecan (T-DXd; DS-8201) in patients (pts) with HER2-expressing unresectable or recurrent biliary tract cancer (BTC): An investigator-initiated multicenter phase 2 study (HERB trial). JCO 2022;40:4006.
30. Harding JJ, Fan J, Oh DY, et al. HERIZON-BTC-01 study group. Zanidatamab for HER2-amplified, unresectable, locally advanced or metastatic biliary tract cancer (HERIZON-BTC-01): a multicentre, single-arm, phase 2b study. Lancet Oncol 2023;24:772-82.
31. Harding JJ, Lowery MA, Shih AH, et al. Isoform switching as a mechanism of acquired resistance to mutant isocitrate dehydrogenase inhibition. Cancer Discov 2018;8:1540-7.
32. Cleary JM, Rouaisnel B, Daina A, et al. Secondary IDH1 resistance mutations and oncogenic IDH2 mutations cause acquired resistance to ivosidenib in cholangiocarcinoma. NPJ Precis Oncol 2022;6:61.
33. Ornitz DM, Itoh N. The fibroblast growth factor signaling pathway. Wiley Interdiscip Rev Dev Biol 2015;4:215-66.
35. Silverman IM, Murugesan K, Lihou CF, et al. Comprehensive genomic profiling in FIGHT-202 reveals the landscape of actionable alterations in advanced cholangiocarcinoma. JCO 2019;37:4080.
36. Javle MM, Murugesan K, Shroff RT, et al. Profiling of 3,634 cholangiocarcinomas (CCA) to identify genomic alterations (GA), tumor mutational burden (TMB), and genomic loss of heterozygosity (gLOH). JCO 2019;37:4087.
37. Meric-Bernstam F, Bahleda R, Hierro C, et al. Futibatinib, an irreversible FGFR1-4 inhibitor, in patients with advanced solid tumors harboring FGF/FGFR aberrations: a phase I dose-expansion study. Cancer Discov 2022;12:402-15.
38. Goyal L, Kongpetch S, Crolley VE, Bridgewater J. Targeting FGFR inhibition in cholangiocarcinoma. Cancer Treat Rev 2021;95:102170.
39. Farha N, Dima D, Ullah F, Kamath S. Precision oncology targets in biliary tract cancer. Cancers 2023;15:2105.
40. FDA grants accelerated approval to pemigatinib for cholangiocarcinoma with an FGFR2 rearrangement or fusion. Available from: https://www.fda.gov/drugs/resources-information-approved-drugs/fda-grants-accelerated-approval-pemigatinib-cholangiocarcinoma-fgfr2-rearrangement-or-fusion [Last accessed on 25 Dec 2023].
41. Abou-Alfa GK, Sahai V, Hollebecque A, et al. Pemigatinib for previously treated, locally advanced or metastatic cholangiocarcinoma: a multicentre, open-label, phase 2 study. Lancet Oncol 2020;21:671-84.
42. Vogel A, Sahai V, Hollebecque A, et al. O-2 Pemigatinib for previously treated locally advanced or metastatic cholangiocarcinoma: Final results from FIGHT-202. Ann Oncol 2022;33:S379.
43. Bekaii-Saab TS, Valle JW, Van Cutsem E, et al. FIGHT-302: first-line pemigatinib vs gemcitabine plus cisplatin for advanced cholangiocarcinoma with FGFR2 rearrangements. Future Oncol 2020;16:2385-99.
44. FDA grants accelerated approval to futibatinib for cholangiocarcinoma. Available from: https://www.fda.gov/drugs/resources-information-approved-drugs/fda-grants-accelerated-approval-futibatinib-cholangiocarcinoma [Last accessed on 25 Dec 2023].
45. Goyal L, Meric-Bernstam F, Hollebecque A, et al. FOENIX-CCA2 Study Investigators. Futibatinib for FGFR2-rearranged intrahepatic cholangiocarcinoma. N Engl J Med 2023;388:228-39.
46. Goyal L, Meric-bernstam F, Hollebecque A, et al. Updated results of the FOENIX-CCA2 trial: Efficacy and safety of futibatinib in intrahepatic cholangiocarcinoma (iCCA) harboring FGFR2 fusions/rearrangements. JCO 2022;40:4009.
47. Lau DK, Jenkins L, Weickhardt A. Mechanisms of acquired resistance to fibroblast growth factor receptor targeted therapy. Cancer Drug Resist 2019;2:568-79.
48. Schaider H, Sturm R. The evolving universe of BRAF mutations in melanoma. Br J Dermatol 2017;177:893.
49. Rose AAN. Encorafenib and binimetinib for the treatment of BRAF V600E/K-mutated melanoma. Drugs Today 2019;55:247-64.
50. Caputo F, Santini C, Bardasi C, et al. BRAF-mutated colorectal cancer: clinical and molecular insights. Int J Mol Sci 2019;20:5369.
51. Ritterhouse LL, Barletta JA. BRAF V600E mutation-specific antibody: A review. Semin Diagn Pathol 2015;32:400-8.
52. Subbiah V, Lassen U, Élez E, et al. Dabrafenib plus trametinib in patients with BRAFV600E-mutated biliary tract cancer (ROAR): a phase 2, open-label, single-arm, multicentre basket trial. Lancet Oncol 2020;21:1234-43.
53. FDA grants accelerated approval to dabrafenib in combination with trametinib for unresectable or metastatic solid tumors with BRAF V600E mutation. Available from: https://www.fda.gov/drugs/resources-information-approved-drugs/fda-grants-accelerated-approval-dabrafenib-combination-trametinib-unresectable-or-metastatic-solid [Last accessed on 25 Dec 2023].
54. Petitjean A, Achatz MI, Borresen-Dale AL, Hainaut P, Olivier M. TP53 mutations in human cancers: functional selection and impact on cancer prognosis and outcomes. Oncogene 2007;26:2157-65.
55. Rizos H, Menzies AM, Pupo GM, et al. BRAF inhibitor resistance mechanisms in metastatic melanoma: spectrum and clinical impact. Clin Cancer Res 2014;20:1965-77.
56. Shi H, Hugo W, Kong X, et al. Acquired resistance and clonal evolution in melanoma during BRAF inhibitor therapy. Cancer Discov 2014;4:80-93.
57. Van Allen EM, Wagle N, Sucker A, et al; Dermatologic Cooperative Oncology Group of Germany (DeCOG). The genetic landscape of clinical resistance to RAF inhibition in metastatic melanoma. Cancer Discov 2014;4:94-109.
58. Shi H, Moriceau G, Kong X, et al. Melanoma whole-exome sequencing identifies (V600E)B-RAF amplification-mediated acquired B-RAF inhibitor resistance. Nat Commun 2012;3:724.
59. Wagle N, Emery C, Berger MF, et al. Dissecting therapeutic resistance to RAF inhibition in melanoma by tumor genomic profiling. J Clin Oncol 2011;29:3085-96.
60. Long GV, Fung C, Menzies AM, et al. Increased MAPK reactivation in early resistance to dabrafenib/trametinib combination therapy of BRAF-mutant metastatic melanoma. Nat Commun 2014;5:5694.
61. Wang B, Zhang W, Zhang G, et al. Targeting mTOR signaling overcomes acquired resistance to combined BRAF and MEK inhibition in BRAF-mutant melanoma. Oncogene 2021;40:5590-9.
62. Pópulo H, Lopes JM, Soares P. The mTOR signalling pathway in human cancer. Int J Mol Sci 2012;13:1886-918.
64. Iqbal N, Iqbal N. Human epidermal growth factor receptor 2 (HER2) in cancers: overexpression and therapeutic implications. Mol Biol Int 2014;2014:852748.
65. Ayasun R, Ozer M, Sahin I. The role of HER2 status in the biliary tract cancers. Cancers 2023;15:2628.
66. Galdy S, Lamarca A, McNamara MG, et al. HER2/HER3 pathway in biliary tract malignancies; systematic review and meta-analysis: a potential therapeutic target? Cancer Metastasis Rev 2017;36:141-57.
67. Varga Z, Noske A, Ramach C, Padberg B, Moch H. Assessment of HER2 status in breast cancer: overall positivity rate and accuracy by fluorescence in situ hybridization and immunohistochemistry in a single institution over 12 years: a quality control study. BMC Cancer 2013;13:615.
68. Junttila TT, Akita RW, Parsons K, et al. Ligand-independent HER2/HER3/PI3K complex is disrupted by trastuzumab and is effectively inhibited by the PI3K inhibitor GDC-0941. Cancer Cell 2009;15:429-40.
69. Javle M, Borad MJ, Azad NS, et al. Pertuzumab and trastuzumab for HER2-positive, metastatic biliary tract cancer (MyPathway): a multicentre, open-label, phase 2a, multiple basket study. Lancet Oncol 2021;22:1290-300.
70. Tsurutani J, Iwata H, Krop I, et al. Targeting HER2 with trastuzumab deruxtecan: a dose-expansion, phase i study in multiple advanced solid tumors. Cancer Discov 2020;10:688-701.
71. Vernieri C, Milano M, Brambilla M, et al. Resistance mechanisms to anti-HER2 therapies in HER2-positive breast cancer: current knowledge, new research directions and therapeutic perspectives. Crit Rev Oncol Hematol 2019;139:53-66.
72. Zhao D, Klempner SJ, Chao J. Progress and challenges in HER2-positive gastroesophageal adenocarcinoma. J Hematol Oncol 2019;12:50.
73. Chen C, Di Bartolomeo M, Corallo S, Strickler JH, Goyal L. Overcoming resistance to targeted therapies in gastrointestinal cancers: progress to date and progress to come. Am Soc Clin Oncol Educ Book 2020;40:161-73.
74. Kwak EL, Ahronian LG, Siravegna G, et al. Molecular heterogeneity and receptor coamplification drive resistance to targeted therapy in MET-amplified esophagogastric cancer. Cancer Discov 2015;5:1271-81.
75. Pietrantonio F, Caporale M, Morano F, et al. HER2 loss in HER2-positive gastric or gastroesophageal cancer after trastuzumab therapy: Implication for further clinical research. Int J Cancer 2016;139:2859-64.
76. Seo S, Ryu MH, Park YS, et al. Loss of HER2 positivity after anti-HER2 chemotherapy in HER2-positive gastric cancer patients: results of the GASTric cancer HER2 reassessment study 3 (GASTHER3). Gastric Cancer 2019;22:527-35.
77. Harding JJ, Piha-Paul SA, Shah RH, et al. Antitumour activity of neratinib in patients with HER2-mutant advanced biliary tract cancers. Nat Commun 2023;14:630.
78. Vikis HG, Clark O, Furegato M, et al. Mapping the epidemiologic characteristics of cancer patients with NTRK gene fusions: a real-world analysis of the cerner enviza eletronic health records (EHR) database. JCO 2022;40:e15123.
79. U.S. Food & Drug Administration. FDA Approves larotrectinib for solid tumors with NTRK gene fusions. Available from: https://www.fda.gov/drugs/fda-approves-larotrectinib-solid-tumors-ntrk-gene-fusions [Last accessed on 25 Dec 2023].
80. U.S. Food & Drug Administration. FDA approves entrectinib for NTRK solid tumors and ROS-1 NSCLC. Available from: https://www.fda.gov/drugs/resources-information-approved-drugs/fda-approves-entrectinib-ntrk-solid-tumors-and-ros-1-nsclc [Last accessed on 25 Dec 2023].
81. Drilon AE, Hong DS, van Tilburg CM, et al. Long-term efficacy and safety of larotrectinib in a pooled analysis of patients with tropomyosin receptor kinase (TRK) fusion cancer. JCO 2022;40:3100.
82. Doebele RC, Drilon A, Paz-Ares L, et al. trial investigators. Entrectinib in patients with advanced or metastatic NTRK fusion-positive solid tumours: integrated analysis of three phase 1-2 trials. Lancet Oncol 2020;21:271-82.
83. Krzakowski MJ, Lu S, Cousin S, et al. Updated analysis of the efficacy and safety of entrectinib in patients (pts) with locally advanced/metastatic NTRK fusion-positive ( NTRK -fp) solid tumors. JCO 2022;40:3099.
84. Kojadinovic A, Laderian B, Mundi PS. Targeting TRK: A fast-tracked application of precision oncology and future directions. Crit Rev Oncol Hematol 2021;165:103451.
85. Harada G, Choudhury NJ, Schram AM, et al. Mechanisms of acquired resistance to TRK inhibitors. JCO 2022;40:3104.
86. Katayama R, Gong B, Togashi N, et al. The new-generation selective ROS1/NTRK inhibitor DS-6051b overcomes crizotinib resistant ROS1-G2032R mutation in preclinical models. Nat Commun 2019;10:3604.
87. Paratala BS, Chung JH, Williams CB, et al. RET rearrangements are actionable alterations in breast cancer. Nat Commun 2018;9:4821.
88. Drusbosky LM, Rodriguez E, Dawar R, Ikpeazu CV. Therapeutic strategies in RET gene rearranged non-small cell lung cancer. J Hematol Oncol 2021;14:50.
89. Kato S, Subbiah V, Marchlik E, Elkin SK, Carter JL, Kurzrock R. RET aberrations in diverse cancers: next-generation sequencing of 4,871 patients. Clin Cancer Res 2017;23:1988-97.
90. Subbiah V, Cassier PA, Siena S, et al. Pan-cancer efficacy of pralsetinib in patients with RET fusion-positive solid tumors from the phase 1/2 ARROW trial. Nat Med 2022;28:1640-5.
91. FDA approves pralsetinib for lung cancer with RET gene fusions. Available from: https://www.fda.gov/drugs/resources-information-approved-drugs/fda-approves-pralsetinib-lung-cancer-ret-gene-fusions [Last accessed on 25 Dec 2023].
92. FDA approves pralsetinib for RET-altered thyroid cancers. Available from: https://www.fda.gov/drugs/resources-information-approved-drugs/fda-approves-pralsetinib-ret-altered-thyroid-cancers#:~:text=On%20December%201%2C%202020%2C%20the,fusion%2Dpositive%20thyroid%20cancer%20who [Last accessed on 25 Dec 2023].
93. FDA D.I.S.C.O. Burst Edition: FDA approvals of Retevmo (selpercatinib) for adult patients with locally advanced or metastatic RET fusion-positive solid tumors, and Retevmo (selpercatinib) for adult patients with locally advanced or metastatic RET fusion-positive non-small cell lung cancer. Available from: https://www.fda.gov/drugs/resources-information-approved-drugs/fda-disco-burst-edition-fda-approvals-retevmo-selpercatinib-adult-patients-locally-advanced-or#:~:text=On%20September%2021%2C%202022%2C%20the,who%20have%20no%20satisfactory%20alternative [Last accessed on 25 Dec 2023].
94. Subbiah V, Wolf J, Konda B, et al. Tumour-agnostic efficacy and safety of selpercatinib in patients with RET fusion-positive solid tumours other than lung or thyroid tumours (LIBRETTO-001): a phase 1/2, open-label, basket trial. Lancet Oncol 2022;23:1261-73.
95. Santoro M, Moccia M, Federico G, Carlomagno F. RET gene fusions in malignancies of the thyroid and other tissues. Genes 2020;11:424.
96. Andrini E, Mosca M, Galvani L, et al. Non-small-cell lung cancer: how to manage RET-positive disease. Drugs Context 2022;11:1-12.
97. Rosen EY, Won HH, Zheng Y, et al. The evolution of RET inhibitor resistance in RET-driven lung and thyroid cancers. Nat Commun 2022;13:1450.
98. Cha JH, Chan LC, Li CW, Hsu JL, Hung MC. Mechanisms controlling PD-L1 expression in cancer. Mol Cell 2019;76:359-70.
99. Riaz N, Havel JJ, Makarov V, et al. Tumor and microenvironment evolution during immunotherapy with nivolumab. Cell 2017;171:934-49.e16.
100. Rizvi H, Sanchez-Vega F, La K, et al. Molecular determinants of response to anti-programmed cell death (PD)-1 and anti-programmed death-ligand 1 (PD-L1) blockade in patients with non-small-cell lung cancer profiled with targeted next-generation sequencing. J Clin Oncol 2018;36:633-41.
102. FDA approves pembrolizumab for adults and children with TMB-H solid tumors. Available from: https://www.fda.gov/drugs/drug-approvals-and-databases/fda-approves-pembrolizumab-adults-and-children-tmb-h-solid-tumors [Last accessed on 26 Jan 2024].
103. Marabelle A, Fakih M, Lopez J, et al. Association of tumour mutational burden with outcomes in patients with advanced solid tumours treated with pembrolizumab: prospective biomarker analysis of the multicohort, open-label, phase 2 KEYNOTE-158 study. Lancet Oncol 2020;21:1353-65.
104. Schenker M, Burotto M, Richardet M, et al. Abstract CT022: CheckMate 848: A randomized, open-label, phase 2 study of nivolumab in combination with ipilimumab or nivolumab monotherapy in patients with advanced or metastatic solid tumors of high tumor mutational burden. Cancer Research 2022;82:CT022.
105. Li K, Luo H, Huang L, Luo H, Zhu X. Microsatellite instability: a review of what the oncologist should know. Cancer Cell Int 2020;20:16.
106. FDA Converts to Full Approval Indication for KEYTRUDA® (pembrolizumab) for Certain Adult and Pediatric Patients With Advanced Microsatellite Instability-High (MSI-H) or Mismatch Repair Deficient (dMMR) Solid Tumors. Available from: https://www.merck.com/news/fda-converts-to-full-approval-indication-for-keytruda-pembrolizumab-for-certain-adult-and-pediatric-patients-with-advanced-microsatellite-instability-high-msi-h-or-mismatch-repair-deficient/ [Last accessed on 25 Dec 2023].
107. Andre T, Berton D, Curigliano G, et al. Safety and efficacy of anti–PD-1 antibody dostarlimab in patients (pts) with mismatch repair-deficient (dMMR) solid cancers: Results from GARNET study. JCO 2021;39:9.
108. FDA grants accelerated approval to dostarlimab-gxly for dMMR advanced solid tumors. Available from: https://www.fda.gov/drugs/resources-information-approved-drugs/fda-grants-accelerated-approval-dostarlimab-gxly-dmmr-advanced-solid-tumors [Last accessed on 25 Dec 2023].
109. Sangsuwannukul T, Supimon K, Sujjitjoon J, et al. Anti-tumour effect of the fourth-generation chimeric antigen receptor T cells targeting CD133 against cholangiocarcinoma cells. Int Immunopharmacol 2020;89:107069.
110. Feng KC, Guo YL, Liu Y, et al. Cocktail treatment with EGFR-specific and CD133-specific chimeric antigen receptor-modified T cells in a patient with advanced cholangiocarcinoma. J Hematol Oncol 2017;10:4.
111. Guo Y, Feng K, Liu Y, et al. Phase I study of chimeric antigen receptor-modified T Cells in patients with EGFR-positive advanced biliary tract cancers. Clin Cancer Res 2018;24:1277-86.
112. Hassan R, Butler MO, Oh DY, et al. Phase 1 trial of gavocabtagene autoleucel (gavo-cel, TC-210) in patients (pts) with treatment refractory mesothelioma and other mesothelin-expressing solid tumors. JCO 2023;41:8537-8537.
113. Morash M, Mitchell H, Beltran H, Elemento O, Pathak J. The role of next-generation sequencing in precision medicine: a review of outcomes in oncology. J Pers Med 2018;8:30.
114. Ramón Y Cajal S, Sesé M, Capdevila C, et al. Clinical implications of intratumor heterogeneity: challenges and opportunities. J Mol Med 2020;98:161-77.
115. Wu P, Gao W, Su M, et al. Adaptive mechanisms of tumor therapy resistance driven by tumor microenvironment. Front Cell Dev Biol 2021;9:641469.
116. Nong S, Han X, Xiang Y, et al. Metabolic reprogramming in cancer: Mechanisms and therapeutics. MedComm 2023;4:e218.
117. Sabnis AJ, Bivona TG. Principles of resistance to targeted cancer therapy: lessons from basic and translational cancer biology. Trends Mol Med 2019;25:185-97.
118. Carpizo DR, D'Angelica M. Management and extent of resection for intrahepatic cholangiocarcinoma. Surg Oncol Clin N Am 2009;18:289-305, viii.
119. Buettner S, Koerkamp BG, Ejaz A, et al. The effect of preoperative chemotherapy treatment in surgically treated intrahepatic cholangiocarcinoma patients-a multi-institutional analysis. J Surg Oncol 2017;115:312-8.
120. Mason MC, Massarweh NN, Tzeng CD, et al. Time to rethink upfront surgery for resectable intrahepatic cholangiocarcinoma? Implications from the neoadjuvant experience. Ann Surg Oncol 2021;28:6725-35.
121. Utuama O, Permuth JB, Dagne G, et al. Neoadjuvant chemotherapy for intrahepatic cholangiocarcinoma: a propensity score survival analysis supporting use in patients with high-risk disease. Ann Surg Oncol 2021;28:1939-49.
122. Sutton TL, Billingsley KG, Walker BS, et al. Neoadjuvant chemotherapy is associated with improved survival in patients undergoing hepatic resection for intrahepatic cholangiocarcinoma. Am J Surg 2021;221:1182-7.
123. Preoperative nab-paclitaxel, cisplatin, and gemcitabine chemotherapy with or without infigratinib targeted therapy for the treatment of resectable intrahepatic cholangiocarcinoma, the OPTIC trial. Available from: https://clinicaltrials.gov/study/NCT05514912?cond=targeted%20neoadjuvant%20cholangiocarcinoma&rank=1 [Last accessed on 25 Dec 2023].
Cite This Article
Export citation file: BibTeX | RIS
OAE Style
Gim G, Badri N. Challenges and opportunities for treating intrahepatic cholangiocarcinoma: targeted therapy. Hepatoma Res 2023;9:51. http://dx.doi.org/10.20517/2394-5079.2023.90
AMA Style
Gim G, Badri N. Challenges and opportunities for treating intrahepatic cholangiocarcinoma: targeted therapy. Hepatoma Research. 2023; 9: 51. http://dx.doi.org/10.20517/2394-5079.2023.90
Chicago/Turabian Style
Gim, Gahyun, Nabeel Badri. 2023. "Challenges and opportunities for treating intrahepatic cholangiocarcinoma: targeted therapy" Hepatoma Research. 9: 51. http://dx.doi.org/10.20517/2394-5079.2023.90
ACS Style
Gim, G.; Badri N. Challenges and opportunities for treating intrahepatic cholangiocarcinoma: targeted therapy. Hepatoma. Res. 2023, 9, 51. http://dx.doi.org/10.20517/2394-5079.2023.90
About This Article
Special Issue
Copyright
Data & Comments
Data
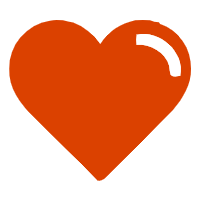

Comments
Comments must be written in English. Spam, offensive content, impersonation, and private information will not be permitted. If any comment is reported and identified as inappropriate content by OAE staff, the comment will be removed without notice. If you have any queries or need any help, please contact us at support@oaepublish.com.