Review of IDH mutations and potential therapies for intrahepatic cholangiocarcinoma
Abstract
Cholangiocarcinoma (CCA) is an aggressive malignancy that arises from the biliary tract. Currently, the first-line therapy for advanced CCA is gemcitabine and cisplatin. However, 5-year survival remains low. It has become abundantly clear that a “one size fits all” approach no longer applies to the treatment of individual cancers, given the large amount of tumor heterogeneity. As such, research in recent years has focused on developing effective targeted therapies through genetic profiling of CCA tumors. IDH1 and IDH2 mutations are commonly found in intrahepatic CCA (ICCA). IDH mutations prevent hepatic progenitor cell differentiation and result in the persistence of progenitor-like and stem cells. These are more prone to alterations that promote tumor initiation. As such, IDH has been identified as a promising target for ICCA treatment. We herein review the role of IDH mutations in ICCA development, recent data for IDH inhibitors in ICCA treatment, and challenges within the field of targeted therapy for ICCA.
Keywords
INTRODUCTION
Cholangiocarcinoma (CCA) is a rare and aggressive malignancy that originates from the biliary tract. It can be divided anatomically into intrahepatic and extrahepatic (hilar and distal) CCA. Intrahepatic CCA (ICCA) originates from the second-order bile ducts in the liver and is thought to differ from extrahepatic CCA (ECCA) in underlying biology and behavior[1]. Surgical resection with adjuvant capecitabine carries the best hope of achieving long-term survival. Given the propensity to develop recurrent or metastatic disease, patients who undergo curative intent surgery still only have a 5-year survival rate between
Based on results from the ABC-02 trial, the combination of gemcitabine and cisplatin is currently the
Genetic profiling of CCA tumors has started to identify common mutations that can be targeted. Javle et al. performed next-generation sequencing on 554 biliary tract cancers (BTC), including 412 ICCA, and reported the most common genetic aberrations[11]. The most common mutations in patients with ICCA were cyclin-dependent kinase inhibitor 2A/B (CDKN2A/B; 27%), tumor protein 53 (TP53; 27%), KRAS (22%), AT-rich interactive domain-containing protein 1A (ARID1A; 18%), and isocitrate dehydrogenase 1 (IDH1; 16%). Weinberg et al. profiled 1,502 BTC (825 were ICCA) and found a higher rate of IDH1, ubiquitin carboxyl-terminal hydrolase (BAP1), polybromo-1 (PBRM1), and fibroblast growth factor receptor (FGFR) genetic aberrations in ICCA tumors[12]. IDH1 has been identified as a promising target for ICCA treatment. We herein review the role IDH mutations play in ICCA development, recent data for IDH inhibitors in ICCA treatment, and the challenges within the field of targeted therapy for ICCA.
MOLECULAR PATHOGENESIS OF INTRAHEPATIC CHOLANGIOCARCINOMA
ICCA originates from the second-order bile ducts within the liver when the epithelial cells lining the bile duct undergo malignant transformation. The molecular mechanism of cholangiocarcinoma has not been completely elucidated. A persistent pro-inflammatory state combined with increased growth factors and bile acids may lead to the accumulation of mutations in cholangiocytes and uncontrolled cell growth. The delicate homeostasis of the liver’s immunotolerant microenvironment is disrupted, leading to oncogenesis[13]. Sia et al. found two transcriptomic profiles of ICCA through gene expression profiling: the “inflammation” subtype and the “proliferation” subtype[14]. In the inflammation subtype, there is activation of immune-related signaling pathways and an overexpression of cytokines. In contrast, the proliferation subtype is described as activation of oncogenic signaling pathways (e.g., RAS, MAPK, MET) and mutations in KRAS and BRAF.
IDH1 molecular pathway
IDH in physiologic conditions
IDH exists in three isoforms: IDH1, IDH2, and IDH3. IDH1 expression is highest in hepatocytes, where it plays a role in lipogenesis and maintenance of redox homeostasis. IDH2 expression is highest in cardiac muscle cells but still expressed in hepatocytes[15]. While mutations in IDH1 and IDH2 genes have been found in 16% and 4% of patients with ICCA, respectively, they are not commonly seen in patients with ECCA[11,16,17]. IDH1 is found in the cytosol, while IDH2 is found in the mitochondria[18]. In physiologic conditions, IDH1 and IDH2 enzymes participate in a two-step reaction in the Krebs cycle that converts isocitrate to α-ketoglutarate (α-KG). In the first part of the reaction, isocitrate is converted through oxidation to oxalosuccinate. Subsequently, the beta-carbonyl group on oxalosuccinate is released as CO2 to form α-KG. This reaction also results in the reduction of two NADP+ molecules to NADPH[19,20] [Figure 1]. This reduction supports the scavenging of reactive oxygen species and cholesterol biosynthesis. Additionally, α-KG acts as a co-substrate for many enzymes. When cells are exposed to hypoxia, IDH1 and IDH2 can reverse the reaction and convert α-KG back to isocitrate and replenish the Krebs cycle or generate acetyl-CoA[15].
Figure 1. IDH1 and IDH2 mutations causes build up of 2-hydroxygluatrate (2-HG). IDH: isocitrate dehydrogenase; CO2: Carbon dioxide; NADP+: nicotinamide adenine dinucleotide phosphate; NADPH: reduced form of NADP. This figure was re-printed from open access reference[20].
Role of IDH mutations in ICCA
Most commonly, IDH1 and IDH2 mutations are secondary to a point mutation in the R132 and R172 codons, respectively[21]. The pathways for how mutated IDH leads to oncogenesis are still poorly understood, but pieces of the puzzle have been elucidated through various studies. There is general consensus that the primary oncogenic mechanism of IDH mutations is through an accumulation of 2-hydroxyglutarate (2-HG)[15] [Figure 1]. Gain of function mutations in IDH1 or IDH2 results in the generation of 2-hydroxyglutarate (2-HG) and a decrease in α-KG and NADPH. 2-HG is structurally similar to α-KG and competitively binds and inhibits dioxygenase enzymes[15,18,20]. These enzymes include regulators of cell differentiation and metabolism[22]. In addition, the decreased levels of α-KG results in the degradation of hypoxia-inducible factor 1α (HIF-1α) and increased angiogenesis, while the reduction in NADPH leads to an increase in reactive oxygen species[18,22]. Through these various mechanisms, IDH mutations prevent hepatic progenitor cell differentiation and result in the persistence of stem and progenitor-like cells. These cells are more prone to oncogenic alterations that promote tumor initiation[15].
In recent years, the immune tumor microenvironment has emerged as a crucial component of tumor development and progression. Some studies have demonstrated that IDH mutations play a role in immune modulation of the microenvironment. Studies in gliomas have demonstrated an association between IDH1 mutations and low intra-tumoral CD8+ T cells and immune-related signaling compared to IDH1 wild-type tumors[23,24]. The increased presence of 2-HG also contributes to immunosuppression within the tumor microenvironment. In vitro studies have shown that 2-HG is taken up by T-cells. This leads to inhibition of T cell proliferation and reduced production of interferon-γ (IFN-γ) and IL-2. However, it is poorly understood how 2-HG is secreted from tumor cells and then taken up by T cells[15,25].
Most pre-clinical studies for IDH mutations employ pre-clinical models for gliomas or acute myeloid leukemia. Wu et al. utilized pre-clinical models for cholangiocarcinoma to demonstrate that IDH1 mutations resulted in immune suppression through a TET2 inactivation. Additionally, they demonstrated the efficacy of combination therapy with IDH1 inhibitors and immune checkpoint inhibitors (ICI)[26]. Their team created a genetically engineered IDH1 mutated murine model that promoted ICCA development. Biopsies of the mouse livers showed elevated levels of 2-HG and similar histopathologic features of human ICCA. With this model, they found that elevated levels of 2-HG mediated a TET2 inactivation and that IDH1 mutations suppressed anti-tumor immunity by causing an insensitivity of ICCA cells to immune signals and impairing CD8+ T cell function. When an IDH1 inhibitor was employed, the authors saw rapid CD8+ T cell recruitment and decreased tumor growth. Of note, no effect was seen on tumor growth with an IDH1 inhibitor in immunodeficient mice, thereby confirming the necessity of an immune response to observe the anti-tumor effect of IDH1 inhibition. RNA sequencing and immunohistochemistry (IHC) analysis of immunocompetent mice treated with an IDH1 inhibitor showed upregulation of interferon-γ response genes and an increase in CD8+ T cell infiltration.
IDH mutations and prognosis in ICCA
Unfortunately, there is little convincing evidence that the presence of an IDH mutation is associated with prognosis. Wang et al. evaluated 326 patients with resected ICCA and found that IDH mutation was associated with improved overall survival and longer time to tumor recurrence. Tumor recurrence at 1, 4, and 7 years in patients with IDH mutations was 10.5%, 45.3%, and 45.3%, respectively, while recurrence rates in patients with wild-type tumors were 41.7%, 71.5%, and 81.3%, respectively[27]. However, this study did not provide information on tumor stage or other therapies given to the cohort. Rizzato et al. evaluated 286 patients with biliary tract cancers and found on multivariable analysis that IDH mutation was associated with better overall survival[28]. Despite these two studies, there are several more that have not demonstrated any association between IDH mutation and prognosis for ICCA. Zhu et al. performed genomic profiling of 200 ICCA tumors and did not find any significant association between IDH mutation and overall survival[29]. Several other studies have failed to demonstrate an association between IDH mutation and prognosis, even when stratified for competing variables[30-33].
Treatment of IDH-mutated CCA
Ivosidenib
Ivosidenib, also known as AG-120, is an IDH1 inhibitor. It binds mutated IDH1 and locks it in its inactive conformation, thereby allosterically inhibiting the production of 2-HG[34]. It was previously approved for patients with IDH1 mutated acute myeloid leukemia. In a phase I study, ivosidenib monotherapy was tested in IDH1 mutated solid tumors at multiple centers in the United States and France[35]. Included in this study were 73 patients with advanced CCA (65 with ICCA, 8 with ECCA) that had recurred or progressed on standard therapy. Median progression-free survival (PFS) was 3.8 months, with 6- and 12-month PFS reported as 40.1% and 21.8%, respectively. Median overall survival (OS) was 13.8 months. This study demonstrated that ivosidenib is well tolerated in patients with previously treated IDH1 mutated CCA. Additionally, 13 of the patients in this study had post-treatment tumor samples assessed for Ki-67 proliferation index. A reduction in Ki-67 positive cells was seen in 9 of the 13 patients (69%), with a median reduction of 22.6% across all 13 patients.
A recent phase III, randomized, double-blind study compared ivosidenib to placebo in patients with advanced or metastatic IDH1 mutated CCA who have previously received at least one treatment regimen (ClarIDHy, NCT02989857)[33]. They recruited 187 patients and randomized in a 2:1 fashion (ivosidenib vs. placebo). The majority of patients had ICCA (91.4%). Of the 61 patients in the placebo arm, 43 crossed over to the ivosidenib arm. Median OS was 10.3 months and 7.5 months with ivosidenib versus placebo, respectively, but when adjusted for crossover, the median OS for the placebo cohort was 5.1 months
LY3410738
LY3410738 is a selective, covalent inhibitor of IDH1 that binds to a different site of the mutated IDH1 enzyme than ivosidenib. LY3410738 modifies a single cysteine in an allosteric binding pocket and inactivates the enzyme. This inhibits 2-HG production but does not change α-KG levels[37]. Additionally, this binding site is outside of the dimer interface, enabling activity in the setting of known second-site IDH1 mutations[38]. In a pre-clinical study, LY3410738 demonstrated greater potency for inhibition of 2-HG production in vitro compared to ivosidenib. Furthermore, the combination of LY3410738 and a Bcl-2 inhibitor was effective in an acute myeloid leukemia xenograft model that was derived from a patient with ivosidenib resistance[37]. LY3410738 is currently being evaluated in a phase I trial (NCT04521686) in patients with IDH1 mutated solid tumors, including advanced CCA. This study includes patients who had previous treatment with another IDH1 inhibitor. After determining the recommended phase II dose, LY3410738 will be evaluated as a monotherapy and in combination with cisplatin and gemcitabine. The cohort with a combination of LY3410738, gemcitabine, and cisplatin will be in patients with CCA who are
Poly-ADP ribose polymerase inhibitors
Poly-ADP ribose polymerase (PARP) is a family of proteins that are involved in DNA repair. Wang et al. demonstrated with pre-clinical in vitro and in vivo models of gliomas and cholangiocarcinoma that the IDH mutation confers sensitivity to PARP inhibitors[39]. However, this study also suggests that concurrent radiation therapy is necessary to yield the maximum benefit of PARP inhibitors in these patients. Other studies have proposed that the reason IDH-mutated CCA cell lines are sensitive to PARP inhibitors is related to elevated levels of 2-HG[40,41]. Based on these studies, there are ongoing clinical trials evaluating olaparib (PARP inhibitor) in patients with IDH-mutated CCA (NCT03w878095, NCT03212274, NCT03561870, NCT03749187).
Platinum-based chemotherapy
Pre-clinical evidence also indicates that IDH1 and IDH2 mutations result in homologous recombination deficiency. As a result, the cell is unable to effectively repair double-stranded DNA breaks[42]. Some propose that this could be exploited as a target for platinum-based chemotherapy and/or PARP inhibitors. In a retrospective study, Niger et al. investigated whether there was a survival difference with platinum-based therapy in patients with IDH-mutated versus wild-type ICCA[43]. There was no difference in PFS, disease control rate, or overall response rate when comparing patients with IDH-mutated ICCA to patients with wild-type ICCA. In an analysis of the Cancer Genome Atlas CCA tumors, there was no difference in the rate of homologous recombination deficiency between IDH-mutated and wild-type CCA tumors, although CCA did have higher homologous recombination deficiency compared to other tumor types[43]. This suggests that the presence of IDH mutation does not necessarily correlate with elevated homologous recombination deficiency.
Other potential targeted therapies
Dasatinib is a multi-tyrosine kinase inhibitor. One theory is that the changes to cell differentiation, metabolism, and epigenetic control caused by IDH mutations may confer targetable vulnerabilities in other pathways. One pre-clinical study performed a high-throughput drug screen with 17 BTC cell lines. They demonstrated rapid apoptosis in dasatinib-treated, IDH-mutated ICCA cell lines due to a dependency on SRC signaling. SRC is a family of non-receptor tyrosine kinases that are known to play a role in cell proliferation, differentiation, and migration[44]. In the IDH-mutated patient-derived xenografts, dasatinib caused widespread cell necrosis. Of note, levels of SRC expression and activity did not correlate with IDH mutation status or dasatinib sensitivity. In addition, SRC activity was similar in both the IDH wild-type and mutated cell lines. There is currently a phase II trial evaluating the use of dasatinib in patients with IDH-mutated advanced CCA (NCT02428855).
Other pre-clinical studies have demonstrated that the bromodomain and extra-terminal domain (BET) inhibitor JQ1 has an inhibitory effect on IDH-mutated ICCA human cell lines[45]. BET represents a family of proteins that facilitate transcriptional activation through recruitment of transcriptional regulatory complexes[46]. Ongoing clinical trials for IDH-mutated CCA can be found in Table 1.
Selected ongoing clinical trials for IDH-mutated cholangiocarcinoma
Agent | Mechanism | Condition | Study phase | Clinical trial identifier |
Olaparib | PARP Inhibitor | IDH1 or IDH2 mutated, advanced glioma, cholangiocarcinoma, or solid tumors | Phase II | NCT03212274 |
Olaparib and durvalumab | Olaparib: PARP Inhibitor Durvalumab: PD-L1 inhibitor | IDH-mutated glioma or cholangiocarcinoma | Phase II | NCT03991832 |
Olaparib and ceralasertib | Olaparib: PARP inhibitor Ceralasertib: ATR inhibitor | IDH1 and IDH2 mutated cholangiocarcinoma and other solid tumors | Phase II | NCT03878095 |
LY3410738 | IDH1 Inhibitor | IDH1 R132 mutated solid tumors (including cholangiocarcinoma) | Phase I | NCT04521686 |
Dasatinib | Multi-tyrosine kinase inhibitor | IDH-mutated advanced intrahepatic cholangiocarcinoma | Phase II | NCT02428855 |
Ivosidenib and nivolumab | Ivosidenib: IDH1 inhibitor Nivolumab: PD-1 inhibitor | IDH1 mutated gliomas and advanced solid tumors (including cholangiocarcinoma) | Phase II | NCT04056910 |
Ivosidenib or pemigatinib with gemcitabine/cisplatin | Ivosidenib: IDH1 Inhibitor Pemigatinib: FGFR inhibitor | IDH1 mutated or FGFR2 gene aberration cholangiocarcinoma | Phase I | NCT04088188 |
FT-2102 (Olutasidenib) | IDH1 Inhibitor | IDH1 mutated glioma or advanced solid tumors (including cholangiocarcinoma) | Phase I/II | NCT03684811 |
Mechanisms of resistance
Despite the promising future of targeted therapy, a critical hurdle is IDH inhibitor resistance. Resistance can be broken down into primary and secondary (or acquired) resistance. When a tumor does not initially respond to targeted therapy, this is known as primary resistance. On the other hand, secondary resistance occurs when patients demonstrate disease recurrence or progression after an initial response to targeted therapy. Understanding the mechanisms of resistance can guide clinicians as to which targeted therapies will be most effective in patients and how to predict acquired resistance so that treatment regimens can be adjusted.
IDH inhibitor resistance in acute myeloid leukemia
Most studies focused on IDH inhibitor resistance have been in acute myeloid leukemia. In a study of 174 patients with acute myeloid leukemia, the presence of concurrent receptor tyrosine kinase (RTK) pathway mutations was associated with primary resistance to ivosidenib and the development of RTK pathway gene mutations during treatment was associated with secondary resistance. Additionally, restoration of elevated 2-HG levels through acquired second-site IDH1 mutations or new IDH2 mutations was observed as a mechanism of secondary resistance[47]. Wang et al. performed a genomic analysis in longitudinally collected specimens from 60 patients with IDH1 or IDH2 mutated acute myeloid leukemia and treated with IDH inhibitors. They demonstrated that primary resistance to IDH inhibitors was associated with certain
In a study of four patients, mutant IDH isoform switching, either from mutated IDH1 to mutated IDH2 or vice versa, was identified as a mechanism of acquired resistance. Isoform switching re-established elevated 2-HG levels. Three of these patients had acute myeloid leukemia, while the fourth had IDH1 mutated ICCA. In the patient with ICCA, sequencing of the post-progression biopsy after treatment with ivosidenib demonstrated the known IDH1 mutation and a new IDH2 mutation. The patient was then treated with an IDH2 inhibitor, enasidenib, that is approved for acute myeloid leukemia, but unfortunately expired about 2 months after starting treatment[49].
IDH inhibitor resistance in CCA
The data on CCA IDH inhibitor resistance is more limited. Thirty-seven of the patients who progressed on ivosidenib in the phase I trial had paired pre-treatment and post-progression biopsies taken for next-generation sequencing. Of these patients, six had new oncogenic mutations develop at an allele frequency of 5%. Four patients developed co-mutations in the TP53, ARID1A, POLE, PIK3R1, and/or TBX3 genes. One patient with a stable disease prior to progression developed a new IDH1 mutation by converting the R132C allele to an R132F. A second patient who initially had a partial response developed an activating IDH2 mutation[35]. This demonstrates two different mechanisms of resistance. First, similar to other cancers, a change in the IDH1 mutation or gain of IDH2 mutation can promote acquired resistance. Second, the presence of new co-mutations in non-IDH genes may also contribute to secondary resistance.
Cleary et al. described two patients with IDH1 mutated CCA with secondary resistance to ivosidenib[34]. One patient acquired an IDH2 mutation and the other developed a secondary IDH1 mutation. Even though the production of 2-HG is less efficient with the double-mutated IDH1 (R132H/D279N versus R132H), the binding site on the enzyme for ivosidenib is changed. Through an in vitro study, Cleary et al. demonstrated that the double-mutated IDH1 was resistant to ivosidenib. This study also demonstrated in the lab that LY3410738 can successfully bind and inhibit the double-mutated IDH1 enzyme[34].
What these studies demonstrate is that through either pre-existing or new mutations, the tumor is able to maintain elevated 2-HG levels and continue to prevent cell differentiation and drive proliferation. Appropriately identifying co-mutations that confer primary resistance and understanding the underlying mechanism for why these co-mutations result in resistance may elucidate new potential therapeutic targets. Additionally, it will allow clinicians to better select patients who will respond to IDH inhibitor therapy. To overcome acquired resistance, biopsies and genetic analysis of the tumor throughout IDH inhibitor therapy may identify patients who have developed new mutations that will confer secondary resistance prior to progression on clinical scans. This would allow clinicians to either add additional therapies or change the treatment plan. These studies stress the complexity of primary and secondary resistance and that success will likely require combination therapy.
CONCLUSION
ICCA is a rare malignancy that often presents at an advanced stage. Currently, the first-line therapy for advanced CCA is gemcitabine and cisplatin. However, 5-year survival is still extremely low. It has become abundantly clear that a “one size fits all” approach no longer applies to the treatment of individual cancers, considering the large amount of tumor heterogeneity. As such, research in recent years has been motivated to develop effective targeted therapies through genetic profiling of CCA tumors. IDH1 and IDH2 mutations can be found in 16% and 4% of patients with ICCA, respectively. Currently, ivosidenib is approved as second-line therapy for IDH1 mutated CCA. Ongoing clinical trials are currently evaluating the use of other IDH inhibitors that are approved for gliomas or acute myeloid leukemia in patients with advanced CCA.
The main obstacle of targeted therapy is primary and secondary resistance. Overcoming this can only be accomplished through strong translational work. Data from the lab should identify potential targeted therapies, inform clinical trial design, and identify the underlying mechanisms of resistance to better select patients that will benefit the most from specific therapy regimens. Biopsies and genetic analysis of the tumor throughout therapy may identify acquired mutations that confer resistance and inform treatment timelines. Combination therapy is likely the best way to tackle the complexity of the underlying biology of resistance. Given the rarity of these tumors, it is imperative that patients are referred and treated on trial to expedite accrual, increase tissue collection for post-trial genomic analysis, and identify successful therapies. Large institutions and cooperative groups should continue a collaborative effort to test new therapies through clinical trials to maintain forward progress over the next decade.
DECLARATIONS
Authors’ contributionsContributed equally to the design, writing, and editing of this manuscript: Ruff SM, Dillhoff M
Availability of data and materialNot applicable.
Financial support and sponsorshipNone.
Conflicts of interestAll authors declared that there are no conflicts of interest.
Ethical approval and consent to participateNot applicable.
Consent for publicationNot applicable.
Copyright© The Author(s) 2023.
REFERENCES
2. Gad MM, Saad AM, Faisaluddin M, et al. Epidemiology of cholangiocarcinoma; United States incidence and mortality trends. Clin Res Hepatol Gastroenterol 2020;44:885-93.
3. Patel N, Benipal B. Incidence of cholangiocarcinoma in the USA from 2001 to 2015: a US cancer statistics analysis of 50 States. Cureus 2019;11:e3962.
4. Alabraba E, Joshi H, Bird N, et al. Increased multimodality treatment options has improved survival for hepatocellular carcinoma but poor survival for biliary tract cancers remains unchanged. Eur J Surg Oncol 2019;45:1660-7.
5. Valle J, Wasan H, Palmer DH, et al. ABC-02 Trial Investigators. Cisplatin plus gemcitabine versus gemcitabine for biliary tract cancer. N Engl J Med 2010;362:1273-81.
6. Rizvi S, Khan SA, Hallemeier CL, Kelley RK, Gores GJ. Cholangiocarcinoma-evolving concepts and therapeutic strategies. Nat Rev Clin Oncol 2018;15:95-111.
7. Rizzo A, Brandi G. Neoadjuvant therapy for cholangiocarcinoma: a comprehensive literature review. Cancer Treat Res Commun 2021;27:100354.
8. Squires MH, Cloyd JM, Dillhoff M, Schmidt C, Pawlik TM. Challenges of surgical management of intrahepatic cholangiocarcinoma. Expert Rev Gastroenterol Hepatol 2018;12:671-81.
9. Santoni M, Rizzo A, Kucharz J, et al. Complete remissions following immunotherapy or immuno-oncology combinations in cancer patients: the MOUSEION-03 meta-analysis. Cancer Immunol Immun 2023;72:1365-79.
10. Rizzo A, Brandi G. First-line chemotherapy in advanced biliary tract cancer ten years after the ABC-02 trial: "and yet it moves!". Cancer Treat Res Commun 2021;27:100335.
11. Javle M, Bekaii-Saab T, Jain A, et al. Biliary cancer: utility of next-generation sequencing for clinical management. Cancer 2016;122:3838-47.
12. Weinberg BA, Xiu J, Lindberg MR, et al. Molecular profiling of biliary cancers reveals distinct molecular alterations and potential therapeutic targets. J Gastrointest Oncol 2019;10:652-62.
13. Rodrigues PM, Olaizola P, Paiva NA, et al. Pathogenesis of cholangiocarcinoma. Annu Rev Pathol 2021;16:433-63.
14. Sia D, Hoshida Y, Villanueva A, et al. Integrative molecular analysis of intrahepatic cholangiocarcinoma reveals 2 classes that have different outcomes. Gastroenterology 2013;144:829-40.
15. Wu MJ, Shi L, Merritt J, Zhu AX, Bardeesy N. Biology of IDH mutant cholangiocarcinoma. Hepatology 2022;75:1322-37.
16. Valls C, Gumà A, Puig I, et al. Intrahepatic peripheral cholangiocarcinoma: CT evaluation. Abdom Imaging 2000;25:490-6.
17. Seo N, Kim DY, Choi JY. Cross-sectional imaging of intrahepatic cholangiocarcinoma: development, growth, spread, and prognosis. AJR Am J Roentgenol 2017;209:W64-75.
18. Fujii T, Khawaja MR, DiNardo CD, Atkins JT, Janku F. Targeting isocitrate dehydrogenase (IDH) in cancer. Discov Med 2016;21:373-80.
19. Clark O, Yen K, Mellinghoff IK. Molecular pathways: isocitrate dehydrogenase mutations in cancer. Clin Cancer Res 2016;22:1837-42.
20. Rizzo A, Ricci AD, Brandi G. IDH inhibitors in advanced cholangiocarcinoma: Another arrow in the quiver? Cancer Treat Res Commun 2021;27:100356.
21. Zhang W, Zhou H, Wang Y, et al. Systemic treatment of advanced or recurrent biliary tract cancer. Biosci Trends 2020;14:328-41.
22. Brandi G, Rizzo A. IDH inhibitors and immunotherapy for biliary tract cancer: a marriage of convenience? Int J Mol Sci 2022;23:10869.
23. Kohanbash G, Carrera DA, Shrivastav S, et al. Isocitrate dehydrogenase mutations suppress STAT1 and CD8+ T cell accumulation in gliomas. J Clin Invest 2017;127:1425-37.
24. Klemm F, Maas RR, Bowman RL, et al. Interrogation of the microenvironmental landscape in brain tumors reveals disease-specific alterations of immune cells. Cell 2020;181:1643-60.e17.
25. Bunse L, Pusch S, Bunse T, et al. Suppression of antitumor T cell immunity by the oncometabolite (R)-2-hydroxyglutarate. Nat Med 2018;24:1192-203.
26. Wu MJ, Shi L, Dubrot J, et al. Mutant IDH inhibits IFNγ-TET2 signaling to promote immunoevasion and tumor maintenance in cholangiocarcinoma. Cancer Discov 2022;12:812-35.
27. Wang P, Dong Q, Zhang C, et al. Mutations in isocitrate dehydrogenase 1 and 2 occur frequently in intrahepatic cholangiocarcinomas and share hypermethylation targets with glioblastomas. Oncogene 2013;32:3091-100.
28. Rizzato M, Brignola S, Munari G, et al. Prognostic impact of FGFR2/3 alterations in patients with biliary tract cancers receiving systemic chemotherapy: the BITCOIN study. Eur J Cancer 2022;166:165-75.
29. Zhu AX, Borger DR, Kim Y, et al. Genomic profiling of intrahepatic cholangiocarcinoma: refining prognosis and identifying therapeutic targets. Ann Surg Oncol 2014;21:3827-34.
30. Boerner T, Drill E, Pak LM, et al. Genetic Determinants of Outcome in Intrahepatic Cholangiocarcinoma. Hepatology 2021;74:1429-44.
31. Goyal L, Govindan A, Sheth RA, et al. Prognosis and clinicopathologic features of patients with advanced stage isocitrate dehydrogenase (IDH) mutant and IDH wild-type intrahepatic cholangiocarcinoma. Oncologist 2015;20:1019-27.
32. Churi CR, Shroff R, Wang Y, et al. Mutation profiling in cholangiocarcinoma: prognostic and therapeutic implications. PLoS One 2014;9:e115383.
33. Zhu AX, Macarulla T, Javle MM, et al. Final overall survival efficacy results of ivosidenib for patients with advanced cholangiocarcinoma with idh1 mutation: the phase 3 randomized clinical clarIDHy trial. JAMA Oncol 2021;7:1669-77.
34. Cleary JM, Rouaisnel B, Daina A, et al. Secondary IDH1 resistance mutations and oncogenic IDH2 mutations cause acquired resistance to ivosidenib in cholangiocarcinoma. NPJ Precis Oncol 2022;6:61.
35. Lowery MA, Burris HA 3rd, Janku F, et al. Safety and activity of ivosidenib in patients with IDH1-mutant advanced cholangiocarcinoma: a phase 1 study. Lancet Gastroenterol Hepatol 2019;4:711-20.
36. NCCN guidelines version: hepatobiliary cancers. 2022. Available from: https://www.nccn.org/guidelines/guidelines-detail?category=1&id=1438 [Last accessed on 31 Jul 2023].
37. Salama V, Brooks N, Skwarska A, et al. Abstract 6417: LY3410738, a novel inhibitor of mutant IDH1 is more effective than Ivosidenib and potentiates antileukemic activity of standard chemotherapy in preclinical models of acute myeloid leukemia (AML). Cancer Res 2020;80:6417.
38. Pauff JM, Papadopoulos KP, Janku F, et al. A phase I study of LY3410738, a first-in-class covalent inhibitor of mutant IDH1 in cholangiocarcinoma and other advanced solid tumors. J Clin Oncol 2021;39:TPS350.
39. Wang Y, Wild AT, Turcan S, et al. Targeting therapeutic vulnerabilities with PARP inhibition and radiation in IDH-mutant gliomas and cholangiocarcinomas. Sci Adv 2020;6:eaaz3221.
40. Schvartzman JM, Reuter VP, Koche RP, Thompson CB. 2-hydroxyglutarate inhibits MyoD-mediated differentiation by preventing H3K9 demethylation. Proc Natl Acad Sci U S A 2019;116:12851-6.
41. Sharma H. Development of novel therapeutics targeting isocitrate dehydrogenase mutations in cancer. Curr Top Med Chem 2018;18:505-24.
42. Stewart MD, Merino Vega D, Arend RC, et al. Homologous recombination deficiency: concepts, definitions, and assays. Oncologist 2022;27:167-74.
43. Niger M, Nichetti F, Casadei-Gardini A, et al. Platinum sensitivity in patients with IDH1/2 mutated
44. Saha SK, Gordan JD, Kleinstiver BP, et al. Isocitrate dehydrogenase mutations confer dasatinib hypersensitivity and SRC dependence in intrahepatic cholangiocarcinoma. Cancer Discov 2016;6:727-39.
45. Fujiwara H, Tateishi K, Kato H, et al. Isocitrate dehydrogenase 1 mutation sensitizes intrahepatic cholangiocarcinoma to the BET inhibitor JQ1. Cancer Sci 2018;109:3602-10.
47. Choe S, Wang H, DiNardo CD, et al. Molecular mechanisms mediating relapse following ivosidenib monotherapy in IDH1-mutant relapsed or refractory AML. Blood Adv 2020;4:1894-905.
48. Wang F, Morita K, DiNardo CD, et al. Leukemia stemness and co-occurring mutations drive resistance to IDH inhibitors in acute myeloid leukemia. Nat Commun 2021;12:2607.
Cite This Article
Export citation file: BibTeX | RIS
OAE Style
Ruff SM, Dillhoff M. Review of IDH mutations and potential therapies for intrahepatic cholangiocarcinoma. Hepatoma Res 2023;9:37. http://dx.doi.org/10.20517/2394-5079.2023.51
AMA Style
Ruff SM, Dillhoff M. Review of IDH mutations and potential therapies for intrahepatic cholangiocarcinoma. Hepatoma Research. 2023; 9: 37. http://dx.doi.org/10.20517/2394-5079.2023.51
Chicago/Turabian Style
Ruff, Samantha M., Mary Dillhoff. 2023. "Review of IDH mutations and potential therapies for intrahepatic cholangiocarcinoma" Hepatoma Research. 9: 37. http://dx.doi.org/10.20517/2394-5079.2023.51
ACS Style
Ruff, SM.; Dillhoff M. Review of IDH mutations and potential therapies for intrahepatic cholangiocarcinoma. Hepatoma. Res. 2023, 9, 37. http://dx.doi.org/10.20517/2394-5079.2023.51
About This Article
Special Issue
Copyright
Data & Comments
Data
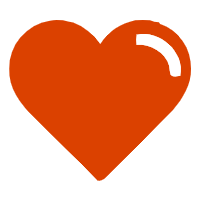

Comments
Comments must be written in English. Spam, offensive content, impersonation, and private information will not be permitted. If any comment is reported and identified as inappropriate content by OAE staff, the comment will be removed without notice. If you have any queries or need any help, please contact us at support@oaepublish.com.