Mutation-based therapies for intrahepatic cholangiocarcinoma: new options on the horizon
Abstract
Intrahepatic cholangiocarcinoma (ICC), a rare but rising global malignancy originating from the bile ducts, poses significant challenges in terms of effective treatment and patient outcomes. While surgical excision remains the curative option, its limited efficacy necessitates more therapeutic strategies, including systemic therapies. The management of ICC involves a multidisciplinary approach, with treatment decisions guided by patient-specific and tumor-specific factors. Gemcitabine-cisplatin (GEMCIS) chemotherapy has been a standard first-line therapy, but recent advancements in immunotherapy, particularly the introduction of durvalumab, have provided new hope. Additionally, gene mutation-based therapies, targeting fibroblast growth factor receptors (FGFRs), isocitrate dehydrogenase-1 (IDH1), human epidermal growth factor receptor-2 (HER2), and B-RAF proto-oncogene (BRAF), offer promising prospects for personalized treatment. High-throughput genomic profiling technologies have facilitated the identification of actionable targets and the development of innovative therapeutic approaches. This review summarizes the mutation-based therapies in ICC, including FDA-approved targeted drugs and ongoing clinical trials, highlighting the evolving landscape of ICC treatment.
Keywords
INTRODUCTION
Cholangiocarcinoma, known as bile duct cancer, is a malignancy originating from the epithelial cells lining the bile ducts. It is a rare and aggressive disease that can develop in various locations along the biliary tract.
In clinical practice, magnetic resonance imaging (MRI) plain scans and dynamic contrast-enhanced scans are commonly employed for the diagnosis of hepatocellular carcinoma (HCC) and cholangiocarcinoma. Specifically, during the arterial phase, hepatocellular carcinoma typically exhibits uniform hypervascularity, accompanied by a significant reduction in enhancement during the portal vein phase, which is lower than that of the normal liver parenchyma[1]. In contrast, ICC manifests as heterogeneous hypervascularity in the arterial phase, with persistent and non-uniform enhancement observed throughout the portal vein and delayed phases, surpassing the enhancement seen in the liver parenchyma[1,2]. It is important to note, however, that at the imaging level, ICC cannot be reliably differentiated from HCC[1]. Categorized into three types based on anatomical location (intrahepatic, perihilar, and distal), cholangiocarcinoma exhibits heterogeneity[3,4]. Among them, intrahepatic cholangiocarcinoma (ICC) exhibits notable heterogeneity, with tumors resembling either conventional mucinous adenocarcinomas (large duct type) or transformed interlobular bile ducts (small duct type)[5]. While the most common risk factors for ICC differ between Western regions, where primary sclerosing cholangitis predominates, and Eastern regions, where parasitic infections are prevalent, the incidence has been similarly increasing in recent years[4,6]. Although radical surgical excision remains the only curative option for ICC, its efficacy is limited. The 5-year post-surgery survival rate for patients is approximately 30%, with a high recurrence rate of 60%-70% within five years post-surgery[7-9]. What is worse, over 70% of patients with ICC are diagnosed with advanced-stage ICC, characterized by either local infiltration or distant metastasis[10,11]. This subgroup of patients has a poor prognosis and does not meet the criteria for surgery, compelling a shift towards systematic therapy[11].
The management of ICC typically relies on patient-specific and tumor-specific factors, and treatment decisions often involve multimodal therapy ideally determined by a multidisciplinary team of experts. This team selects patients for surgical resection, perioperative chemotherapy, transplantation, systemic therapies, and so on. While gemcitabine-cisplatin (GEMCIS) chemotherapy has provided significant relief as a first-line treatment in systemic therapies[12], single GEMICS therapy showed limitations in the coming age of precision medicine. The introduction of immunotherapy has significantly transformed the treatment approach for cholangiocarcinoma over the years, providing renewed hope[10]. One notable advancement is the introduction of durvalumab, a powerful antibody that inhibits the programmed cell death-ligand 1 (PD-L1), into the realm of first-line treatments[13,14]. This therapy has shown significant efficacy and offers new possibilities for patients with advanced ICC. Additionally, in contrast to HCC, the study of gene mutation-based therapies in ICC has progressed rapidly. Several drugs targeting specific gene mutations have received FDA approval as second-line treatment options[15-17]. These options provide a fresh outlook for patients who cannot tolerate first-line chemotherapy or have developed resistance, especially for those with specific gene mutations.
The gold standard for confirming ICC typically involves conducting a pathology examination after a biopsy, and treatment is initiated upon confirmation of the diagnosis. However, given the absence of histologic markers indicating specific biological behavior, such as aggressiveness, the utilization of genetic testing to identify targeted drugs becomes crucial for achieving efficient personalized treatment[18]. The introduction of next-generation sequencing (NGS) has revolutionized genomic profiling technologies, allowing for advanced analysis of genetic information[19], providing valuable insights into initiation, progression, and treatment resistance mechanisms in ICC. These technologies have enabled the identification of specific genetic alterations, unveiling new possibilities for the progression of innovative intervention methods. In ICC, several actionable targets were discovered, with fibroblast growth factor receptors (FGFRs) being of particular interest[10,20,21]. Numerous tyrosine kinase inhibitors targeting FGFRs are currently undergoing assessment in prospective investigations. Moreover, other potential therapeutic targets, including isocitrate dehydrogenase-1 (IDH1)[22,23], human epidermal growth factor receptor-2 (HER2)[24,25], and the BRAF proto-oncogene (BRAF)[25], are also being investigated, showing promising outcomes [Figure 1]. A summary of top mutation targets and corresponding drugs in ICC are listed in Table 1
Top actionable mutation targets and corresponding drugs in ICC
In this review article, we elegantly delineated a series of targeted drugs that were either proved by the FDA or still in clinical trials.
Fibroblast growth factor receptor inhibitors
FGFR2 gene variations are prevalent in ICC, with an incidence ranging from 11% to 45%[17,20,26]. Specifically, FGFR2 rearrangements and aberrations are detected in approximately 15% of ICC cases[27]. In the transformative journey from normal cells to tumor cells in ICC, these genetic mutations play an irreplaceable role by influencing crucial pathways. This impact occurs especially when FGFR2 fusions and rearrangements affect key pathways, ultimately leading to the transition from normal cells to tumor cells[20]. As a result, they have been prospectively assessed and recognized as the targets amenable to intervention[20]. This highlights the potential therapeutic significance of targeting FGFR2 gene variations in ICC management.
Pemigatinib, an FDA-approved selective inhibitor of FGFR2, has demonstrated efficacy in the treatment of ICC with FGFR2 fusion or rearrangement with local infiltration or distant metastasis. The phase II trial, FIGHT-202, provided valuable insights into the effectiveness of pemigatinib in advanced-stage ICC patients[28]. Among those with FGFR2 fusion or rearrangement, the treatment demonstrated a 37.0% objective response rate (ORR) and an 82.4% disease control rate (DCR). The median relapse-free survival (median RFS) and overall survival (OS) were 7.0 months and 17.5 months, respectively, while the median overall survival (median OS) of treated responders reached 30.1 months. Moreover, another study involving 30 subjects assessed the treatment's efficacy[29]. Among them, 15 patients achieved a partial response (PR), resulting in an ORR of 50.0% (95%CI: 31.3-68.7). In addition, it is worth noting that among the 31 patients, a remarkable DCR of 100% (95%CI: 88.4-100) was achieved, with 15 patients demonstrating stable disease (SD). The median time to response was 1.4 months (95%CI: 1.3-1.4), although the duration of response (DOR) remains undetermined. In this study, the median progression-free survival (median PRF) was 6.3 months [95%CI: 4.9-not estimable (NE)]. It is important to mention that out of the total patients, eight individuals (25.8%) experienced grade 3 or higher treatment-emergent adverse events, with the most commonly observed adverse events including hyperphosphatemia, hypophosphatasemia, and so on. These promising findings highlight the significant antitumor activity and positive safety characteristics of pemigatinib, supporting it as a treatment option for patients of ICC with FGFR2 rearrangements. The ongoing phase III randomized trial, FIGHT-302, is presently enlisting participants to investigate and determine the effectiveness of pemigatinib compared to the combination of gemcitabine and cisplatin chemotherapy. This trial focuses on individuals with unresectable or metastatic ICC harboring FGFR2 fusions or rearrangements, serving as a primary treatment option. The overarching goal is to scrutinize and establish the comparative advantages and results of these two distinct treatment modalities[30].
Infigratinib, another FDA-approved FGFR2 inhibitor, has shown promising results in the treatment of advanced ICC with FGFR2 genomic aberrations. A prospective cohort study evaluated infigratinib in 108 patients with advanced ICC, where they received infigratinib (BGJ398)[31,32]. In the subset of individuals with FGFR2 gene fusions or rearrangements (n = 83) in which over half of the patient cohort has received treatments from two or more therapeutic regimens., the ORR, as assessed through centralized review, stands at 23%, accompanied by a median PFS of 7.3 months. Utilized as a second-line intervention, the ORR escalates to 34%, while in the context of third-line and subsequent therapeutic modalities, it diminishes to 13.8%, signifying heightened therapeutic effectiveness when instituting systemic treatment during the advanced stages of the disease. Frequently encountered adverse events during the course of treatment encompass hyperphosphatemia, oral inflammation, and ocular maladies. Given these favorable findings, infigratinib is slated for further examination in a randomized phase III clinical trial denoted as PROOF 301[33]. The PROOF 301 trial aims to provide additional evidence and comparison of infigratinib with other treatment options, further elucidating its efficacy and safety profile.
Futibatinib (TAS-120), a highly selective and irreversible FGFR1-4 inhibitor, has undergone evaluation in phase I and II studies specifically focused on ICC patients with FGFR2 fusions[34,35]. Within the confines of the phase II FOENIX-CCA2 trial, a collective of 103 patients was recruited and subjected to a daily administration of 20 mg of futibatinib. Within this patient cohort, 22% exhibited rearrangements and 78% manifested FGFR2 fusions. The trial results demonstrated an ORR of 43% and a DCR of 85%, with a median DOR of 9.5 months. The median PFS was observed to be 8.9 months, while the median OS reached an impressive 20 months. Adverse effects associated with alternative FGFR inhibitors encompass a range of manifestations, such as hyperphosphatemia and alopecia. Notably, futibatinib has already secured approval from the FDA and will be further assessed in the PHASE III FOENIX-CCA3 TRIAL, specifically focusing on its use as a primary treatment modality for patients diagnosed with ICC and characterized by the presence of documented mutations[36].
Given the effectiveness of targeting FGFR2 fusions in ICC, three distinct randomized phase III trials are underway to compare FGFR inhibitors (pemigatinib, infigratinib, and futibatinib) with gemcitabine and cisplatin chemotherapy[30,33,36].
It provides a concise overview of the trials, medications, and effectiveness associated with the currently available FGFR inhibitors in Table 2.
FGFR inhibitor trials in patients with intrahepatic cholangiocarcinoma
Drug name | ClinicalTrials.gov | Cohort | Test population | Phrase | Findings |
Pemigatinib | FIGHT-202(NCT02924376) | Cohort A: Pemigatinib for subjects with FGFR2 translocation and documented fusion partner. Cohort B: Pemigatinib for subjects with other FGF/FGFR alterations. Cohort C: Pemigatinib for subjects without FGF/FGFR alterations. | Previously treated locally advanced/metastatic CCA harboring FGFR2 fusions/rearrangements | II | ORR: 35.5% DCR: 82% median PFS: 6.9 months (95%CI: 6.2 to 9.6) median OS: 21.1 months (95%CI: 14.8 to Not estimable) |
FIGHT-302(NCT02924376) | Experimental: Pemigatinib Active Comparator: Gemcitabine + Cisplatin | Advanced ICC harboring FGFR2 rearrangements | III | recruiting | |
Infigratinib | CBGJ398X2204(NCT02150967) | Experimental: BGJ398 (infigratinib) | Advanced/metastatic cholangiocarcinoma with FGFR2 translocations | II | ORR: 23.1% (95%CI: 15.6% to 32.2%) DCR: 84.3% (95%CI: 76.0% to 90.6%) median PFS: 7.3 months (95%CI: 5.6 to 7.6) median OS: 12.2 months (95%CI: 10.7 to 14.9) |
Futibatinib | FOENIX-CCA4 (NCT05727176) | Experimental: Futibatinib | Advanced ICC FGFR2 rearrangements | II | ORR: 41.7% median PFS: 8.9 months 12-month OS: 73.1% DCR: 82.5% DOR: 9.5 months 12-month RFS: 35.4% median OS: 20.0 months |
Derazantinib | FIDES-01(NCT03230318) | Experimental: derazantinib | Advanced ICC FGFR2 fusions, rearrangements, or amplification | II | ORR: 21.4% (95%CI: 13.9% to 30.5%) DCR: 75.7% (95%CI: 66.3% to 83.6%) median PFS: 8.0 months (95%CI: 5.5 to 8.3) median OS: 15.9 months (95%CI: 12.5 to 22.6) |
Isocitrate dehydrogenase inhibitors
IDH1 mutations have been identified in a subset of ICC cases, estimated to be approximately 15%-20% of cases[37]. These mutations result in the production of abnormal IDH enzymes that convert isocitrate to an abnormal metabolite, 2-hydroxyglutarate (2-HG), which accumulates in cancer cells and contributes to tumor growth and progression[38,39].
Ivosidenib, a small-molecule inhibitor targeting IDH1 mutant protein, has demonstrated favorable safety and long-term disease stability in patients with ICC[22,40,41]. In 2021, it received approval from the US FDA as a second-line treatment for cholangiocarcinoma patients with local infiltration or distant metastasis who harbor IDH1 mutation. The results of the ClarIDHy trial revealed that the median RFS was 2.7 months in the ivosidenib group compared to 1.4 months in the placebo group[41]. The ORR was 2.4%, with 50.8% of patients experiencing disease stabilization. Additionally, the median OS among patients treated with ivosidenib group was 10.3 months, while it was 7.5 months in the placebo group. In the study, ivosidenib showed a substantial enhancement in PFS compared to placebo, with a PFS of 2.7 months versus 1.4 months, respectively. The hazard ratio was 0.37, indicating a significant reduction in the risk of progression with the P-value, calculated one-sided, less than 0.0001. In a word, the findings revealed that ivosidenib was relatively well-tolerated, and targeting IDH1 mutations in advanced ICC offers notable clinical benefits.
Human epidermal growth factor receptor-2 inhibitors
In contrast to extrahepatic cholangiocarcinoma (ECC), ICC has relatively few mutations in HER2, only 5%-10%[42,43]. Previous studies conducted on ICC patients using lapatinib and varlitinib against HER2 did not produce noteworthy outcomes[44,45]. Nevertheless, it is crucial to emphasize that the lack of HER2 assessment in either drug may have contributed to this outcome. In particular, within pre-selected instances of advanced cholangiocarcinoma exhibiting HER2 genetic modifications, there have been indications of enhanced outcomes.
Neratinib underwent assessment in the SUMMIT trial, which involves diverse histological types[25]. Among the nineteen participants with HER2 mutations who were administered the medication, the median OS was measured in individuals who had previously undergone at least one round of systematic therapy. The observed ORR stood at 10.5%, with a median PFS of 1.8 months.
Additionally, several monoclonal antibody agents targeting HER2 were examined in forward-looking study groups. The amalgamation of trastuzumab and pertuzumab was appraised in the phase II research initiative known as MyPathway[24]. Among the 39 patients with HER2-positive biliary cancer (amplified or overexpressed HER2), nine achieved PR, with an ORR of 23%.
Zanidatamab, a dual-targeting antibody against HER2, was studied in a phase I trial involving 83 patients with tumors exhibiting HER2 amplification, including 22 patients with HER2-expressed biliary tract carcinoma (BTC)[46]. The ORR reached 37% in this study, and there were no deaths attributed to the treatment.
Another experimental medication, trastuzumab deruxtecan (DS-8201), was assessed in the HERB trial[47]. Among the 22 BTC patients who tested positive for HER2, the ORR was recorded as 36.4%. Furthermore, the DCR was 81.8%, and the median PFS was 5.1 months, with an OS of 7.1 months.
The combination of conventional chemotherapy and trastuzumab has also exhibited effectiveness. In a phase II trial carried out in Korea, assessing the use of FOLFOX alongside trastuzumab in individuals diagnosed with HER2-positive conditions, the therapeutic regimen yielded a 29.4% ORR[48].
BRAF proto-oncogene inhibitors
BRAF, a proto-oncogene, exerts a pivotal role in the regulation of cell processes[49]. While BRAF mutations are rare in advanced BTC, accounting for only 1%-5% of actionable findings, they are more prevalent in ICC[27]. Specifically, the BRAF V600E mutation in ICC accounts for 27% of the identified BRAF variants, which leads to activation of the MAPK/ERK signaling pathway, causing uncontrolled cell proliferation and contributing to tumor progression[49].
The phrase II, single-arm study, ROAR, examined 43 previously treated individuals with advanced BTC carrying documented mutations[50]. These individuals underwent combination inhibition of the MEK pathway and the BRAF pathway. The ORR was 47%, with a median PFS of 9 months and a median OS of 14 months. However, around 40% of patients experienced serious adverse events, including elevated γ-glutamyl transferase, fever, reduced white blood cell count, and increased blood pressure.
To evaluate the potential survival advantage of this treatment compared to chemotherapy as an earlier line of systemic therapy, larger studies would be required.
Other mutation-based therapy
One analyzed study included 54 adults with advanced or metastatic solid tumors positive for NTRK fusion from 10 different tumor types and 19 histology types[51,52]. The ORR was notable, with 57% of patients demonstrating a response. Among the responders, 7% achieved complete response (CR) and 50% had PR. The median DOR was estimated to be 10 months, indicating a sustained and meaningful clinical benefit. However, it is essential to acknowledge some limitations of the studies analyzed. The data presented in this summary were based on a specific patient population and may not be generalizable to all patients with NTRK fusion-positive ICC. Further investigations are needed to evaluate the efficacy of these targeted therapies in larger cohorts and diverse patient populations.
Previous studies involving immune checkpoint blockade showed modest response rates of 10%-20% in refractory ICC, but these results did not result in regulatory approval for the treatment[53,54]. However, the TOPAZ-1 trial has reignited enthusiasm in this area[14]. This study compared GEMCIS plus durvalumab versus GEMCIS plus placebo. The trial results revealed that the addition of durvalumab resulted in improved outcomes compared to the placebo group. The addition of durvalumab to GEMCIS resulted in an OS of 12.8 months, which was longer than the OS of 11.5 months for GEMCIS alone (P = 0.021). The median PFS was 7.2 months in the durvalumab arm compared to 5.7 months in the GEMCIS arm (P = 0.001). The ORR was 26.7% in the durvalumab arm and 18.7% in the GEMCIS arm. Although the absolute differences in these survival endpoints are relatively small, the tail of the survival curves suggests that a significant proportion of patients (up to 25%) were alive at 2 years and experienced durable responses, which is unprecedented with traditional cytotoxic therapy.
KRAS has recently gained significant attention for its therapeutic development in multiple kinds of tumors[55-57]. In a cohort study conducted by our team, which involves a collective of 1024 individuals diagnosed with ICC, 14 different subcategories of KRAS mutations were identified, impacting 127 individuals (12.4%)[58]. Among the identified KRAS variants, the most common was G12D, accounting for 55 cases (43.3%). This was subsequently G12V with 25 cases (19.7%), G12C with 9 cases (7.1%), and G13D with 8 cases (6.3%). Being one of the most prevalent driver mutations in cancer, KRAS holds significant importance in the development and advancement of tumors[59,60]. In the past, KRAS has long been considered an "undruggable" target due to its essential role in normal cell functioning and the toxicity associated with inhibiting its activity[59]. Nevertheless, recent advancements have rekindled hope, as the FDA approval of sotorasib for KRAS G12C mutations in non-small cell lung cancer (NSCLC) signifies a breakthrough in KRAS-targeted therapy[61,62]. These achievements have sparked renewed interest in exploring KRAS as a therapeutic target in ICC and offer potential avenues for novel treatment strategies.
A comprehensive overview of the trials, medications, and effectiveness of other inhibitors is provided in Table 3.
Research on other actionable targets harboring in ICC
Mutation gene | Trial | Treatment | Phrase | Findings |
IDH1 | ClarIDHy(NCT02989857) | AG-120(Ivosidenib) vs. placebo | III | ORR: 2.4% vs. 0 median PFS: 2.7 months vs. 1.4 months, P < 0.0001 median OS: 10.8 months vs. 9.7 months, HR = 0.69, 95%CI: 0.44 to 1.10, P = 0.060 |
BRAF | ROAR(NCT02034110) | Dabrafenib | II | ORR: 51% 6-month RRS: 63% (95%CI: 47% to 76%) 12-month OS: 56% (95%CI: 38% to 71%) median OS: 14 months (95%CI: 10 to 33) |
HER2 | DPT02 (NCT04482309) | Trastuzumab deruxtecan (T-DXd) | II | HER2-positive: ORR: 36.4% (90%CI: 19.6% to 56.1%) DCR: 81.8% (95%CI: 59.7% to 94.8%) median PFS: 4.4 months (95%CI: 2.8 to 8.3) median OS: 7.1 months (95%CI: 4.7 to 14.6) HER2-low expression: ORR: 12.5% (1/8) (95%CI: 0.3% to 52.7%) DCR: 75.0% (95%CI: 34.9% to 96.8%) median PFS: 4.2 months (95%CI: 1.3 to 6.2) median OS: 8.9 months (95%CI: 3.0 to 12.8) |
NTRK | STARTRK-1(NCT02097810) | Entrectinib | I | ORR = 57% (95%CI:43.2%~70.8%) median RFS:11.2 mouths (95%CI: 8.0 to 14.9) median OS :21.0 mouths (95%CI: 14.9 to NE) |
DISCUSSION
Writing this review, we addressed certain key targets, target-related medications, and drug-related clinical studies in ICC, and provided an outlook in the field along with a critical analysis of its limitations. In the contemporary era, there have been significant changes in the therapy landscape for advanced-stage ICC with the emergence of mutation-based therapy. Drugs such as pemigatinib and futibatinib have shown enhanced OS in comparison to conventional chemotherapeutic treatments in ICC patients who have received prior treatment, with a median OS of approximately 20 months[30]. Additionally, there is a promising outlook for the combined utilization of mutation-targeted therapy and immunotherapy[39]. Immunotherapy, particularly immune checkpoint inhibitors, has shown remarkable success in ICC by exploiting the immune system's ability to recognize and attack cancer cells[63]. Despite this, further investigation and clinical trials are needed to explore the potential benefits and safety considerations of this combined strategy, which might offer new hope for patients.
Mutation-based therapy in ICC holds promising treatment prospects, but it also presents numerous challenges and limitations. In the first place, clinical trials in the field of mutation-based therapy may encounter various challenges. The rarity of these alterations poses difficulty in identifying eligible patients for recruitment[9,42,43]. Moreover, there is competition among trials for recruiting patients with FGFR2 alterations, further complicating the enrollment process. Additionally, the outdated practice of using chemotherapy alone as a comparative arm in trials may limit the ability to accurately assess the efficacy of FGFR inhibitors. Furthermore, detecting genomic alterations, especially in cases where tissue samples are limited, can be challenging.
To address these challenges, it is essential to implement strategies that enhance patient recruitment and improve the accessibility of sequencing methods. This can be achieved by integrating increased dynamism and decentralization into recruitment and examination. One promising approach is the utilization of circulating cell-free tumor DNA (ctDNA) analysis[64,65]. This method offers an alternative means of identifying and detecting genetic modifications, especially in cases where tissue samples are scarce. Ongoing studies are actively investigating the use of ctDNA as a diagnostic tool, although certain platforms still have limitations in detecting specific fusion events[65].
On another front, the emergence of drug resistance poses a significant challenge in the mutation-based therapy of ICC[66-68]. For FGFR2 inhibitors, several mechanisms of drug resistance have been recognized, with gatekeeper mutations as a prominent example[67]. These mutations sustain FGFR pathway signaling and confer resistance to currently available inhibitors. On the other hand, studies primarily focused on acute myeloid leukemia (AML) have revealed receptor tyrosine kinase (RTK) pathway genes as a cause of resistance against IDH1 inhibitors[69]. However, the mechanism of resistance in IDH mutations in ICC requires further investigation.
By gaining a comprehensive understanding of these mechanisms, novel strategies can be developed to overcome resistance and improve patient outcomes. This may involve the development of new drugs, combination therapies, or alternative treatment approaches tailored to the specific resistance mechanisms identified. The ultimate goal is to maximize the benefits of targeted therapies and optimize their long-term effectiveness in the management of ICC.
The integration of immunotherapy with chemotherapy has emerged as a recent focal point in ICC. In a phase II trial, the investigational combination of nivolumab, gemcitabine, and cisplatin exhibited compelling effectiveness and well-tolerated safety in 32 patients[70]. The cohort included individuals resistant to gemcitabine or cisplatin and chemotherapy-naive patients. Encouragingly, the regimen achieved a noteworthy 55.6% ORR, with 18.6% CR, showcasing notable efficacy even in cases resistant to conventional chemotherapy. The DCR reached an impressive 92.6%. For cohort A, where patients were resistant to standard chemotherapy, one achieved a CR, and one achieved a PR. In the chemotherapy-naive cohort B, 61.9% attained an OR. The median PFS for all patients (cohorts A+B) was a robust 6.1 months, and the median (OS) stood at 8.5 months, accompanied by a notable 33.3% 12-month OS rate. Noteworthy adverse events included thrombocytopenia (56%) and neutropenia (22%). These comprehensive findings underscore the promising potential of nivolumab combined with GEMCIS as an effective and tolerable therapeutic approach for patients with advanced BTC, especially in cases resistant to traditional chemotherapy. Despite promising outcomes in the 32-patient cohort, the relatively modest sample size and a median follow-up of 12.8 months may pose challenges in extrapolating findings to a broader patient spectrum and understanding potential long-term implications. While these findings are encouraging, careful consideration of these limitations underscores the need for future well-controlled studies to substantiate and refine these preliminary observations.
Presently, the realm of new adjuvant and adjunct therapies for ICC lacks involvement in clinical studies with mutation-based targeted treatment[71,72]. This absence can be attributed to two primary factors. Firstly, there is an absence of small-molecule targeted drugs that have transitioned into the forefront of treating unresectable ICC. Unlike chemotherapy approaches like GEMCIS, targeted therapies typically come into play after the establishment of a molecular diagnosis. While clinical trials have investigated the responsiveness of unidentified ICC subtypes to HER2-targeted drugs, the limited patient enrollment and scarcity of associated research impede the widespread acceptance of these treatments as primary therapies. Secondly, the significance of postoperative adjuvant therapy in preventing recurrence introduces the consideration that genetic mutations recurring from primary ICC may have undergone substantial changes. In essence, gene mutation types initially identified through surgical specimens may no longer manifest in recurrent tumors. Instead, these tumors might rely on different gene mutations for progression. This assumption implies that targeted therapy based on prior genetic testing results would lose its specificity. Despite explorations into the molecular subtypes of ICC, a universally accepted viewpoint remains elusive, as acknowledged by the majority of scholars[73-75]. Anticipation surrounds the development of a more refined molecular classification of ICC and the progress of non-invasive methods for detecting genetic mutation profiles. Looking ahead, as the molecular classification of ICC matures and non-invasive methods for detecting genetic mutation profiles advance, targeted therapies for ICC can ascend to a new echelon, potentially paving the way for frontline adjuvant and adjunct therapies.
In summary, we summarized the progress and deficiencies in mutation-based therapies for ICC in this review. Mutation-based therapies have offered innovative treatment options for particular groups of patients and acted as potential members in combination therapy, but they still face certain obstacles. In the age of individualized and precision medicine, further research and clinical practice are needed to advance the field of mutation-based therapies.
DECLARATIONS
Authors’ contributions
Manuscript conception and design: Zhou SL, Zhou J, Fan J, Zhou ZJ
Manuscript draft and revision: Pan SY, Ye YH
Availability of data and materials
Not applicable.
Financial support and sponsorship
This study was jointly supported by the National Natural Science Foundation of China (No. 82173260, No. 81972708, No. 82072681, No. 82003082, No. 81773069, No. 81830102, No. 81772578); the National Key R&D Program of China (No. 2019YFC1315800, 2019YFC1315802, 2018YFA0109400); Shanghai Technical Standard Program (21DZ2201100).
Conflicts of interest
All authors declared that there are no conflicts of interest.
Ethical approval and consent to participate
Not applicable.
Consent for publication
Not applicable.
Copyright
© The Author(s) 2024.
REFERENCES
1. Calderaro J, Ghaffari Laleh N, Zeng Q, et al. Deep learning-based phenotyping reclassifies combined hepatocellular-cholangiocarcinoma. Nat Commun 2023;14:8290.
3. Sung H, Ferlay J, Siegel RL, et al. Global cancer statistics 2020: GLOBOCAN estimates of incidence and mortality worldwide for 36 cancers in 185 countries. CA Cancer J Clin 2021;71:209-49.
4. Morgan E, Arnold M, Gini A, et al. Global burden of colorectal cancer in 2020 and 2040: incidence and mortality estimates from GLOBOCAN. Gut 2023;72:338-44.
5. Labib PL, Goodchild G, Pereira SP. Molecular pathogenesis of cholangiocarcinoma. BMC Cancer 2019;19:185.
6. Khan SA, Tavolari S, Brandi G. Cholangiocarcinoma: epidemiology and risk factors. Liver Int 2019;39 Suppl 1:19-31.
7. Endo I, Gonen M, Yopp AC, et al. Intrahepatic cholangiocarcinoma: rising frequency, improved survival, and determinants of outcome after resection. Ann Surg 2008;248:84-96.
8. Mavros MN, Economopoulos KP, Alexiou VG, Pawlik TM. Treatment and prognosis for patients with intrahepatic cholangiocarcinoma: systematic review and meta-analysis. JAMA Surg 2014;149:565-74.
9. Bertuccio P, Malvezzi M, Carioli G, et al. Global trends in mortality from intrahepatic and extrahepatic cholangiocarcinoma. J Hepatol 2019;71:104-14.
10. Woods E, Le D, Jakka BK, Manne A. Changing landscape of systemic therapy in biliary tract cancer. Cancers 2022;14:2137.
11. Moris D, Palta M, Kim C, Allen PJ, Morse MA, Lidsky ME. Advances in the treatment of intrahepatic cholangiocarcinoma: an overview of the current and future therapeutic landscape for clinicians. CA Cancer J Clin 2023;73:198-222.
12. Valle J, Wasan H, Palmer DH, et al. ABC-02 Trial Investigators. Cisplatin plus gemcitabine versus gemcitabine for biliary tract cancer. N Engl J Med 2010;362:1273-81.
13. Vogel A, Chen L, He AR, et al. Regional subgroup analysis of the phase 3 TOPAZ-1 study of durvalumab (D) plus gemcitabine and cisplatin (GC) in advanced biliary tract cancer (BTC). JCO 2022;40:4075.
14. Oh D, He AR, Qin S, et al. A phase 3 randomized, double-blind, placebo-controlled study of durvalumab in combination with gemcitabine plus cisplatin (GemCis) in patients (pts) with advanced biliary tract cancer (BTC): TOPAZ-1. JCO 2022;40:378.
15. Sia D, Tovar V, Moeini A, Llovet JM. Intrahepatic cholangiocarcinoma: pathogenesis and rationale for molecular therapies. Oncogene 2013;32:4861-70.
16. Saha SK, Gordan JD, Kleinstiver BP, et al. Isocitrate dehydrogenase mutations confer dasatinib hypersensitivity and SRC dependence in intrahepatic cholangiocarcinoma. Cancer Discov 2016;6:727-39.
17. Jusakul A, Cutcutache I, Yong CH, et al. Whole-genome and epigenomic landscapes of etiologically distinct subtypes of cholangiocarcinoma. Cancer Discov 2017;7:1116-35.
18. Desjonqueres E, Campani C, Marra F, Zucman-Rossi J, Nault JC. Preneoplastic lesions in the liver: molecular insights and relevance for clinical practice. Liver Int 2022;42:492-506.
19. van Dijk EL, Auger H, Jaszczyszyn Y, Thermes C. Ten years of next-generation sequencing technology. Trends Genet 2014;30:418-26.
20. De Luca A, Esposito Abate R, Rachiglio AM, et al. FGFR fusions in cancer: from diagnostic approaches to therapeutic intervention. Int J Mol Sci 2020;21:6856.
21. Vogel A, Segatto O, Stenzinger A, Saborowski A. FGFR2 inhibition in cholangiocarcinoma. Annu Rev Med 2023;74:293-306.
22. Abou-Alfa GK, Macarulla T, Javle MM, et al. Ivosidenib in IDH1-mutant, chemotherapy-refractory cholangiocarcinoma (ClarIDHy): a multicentre, randomised, double-blind, placebo-controlled, phase 3 study. Lancet Oncol 2020;21:796-807.
23. Lowery MA, Burris HA 3rd, Janku F, et al. Safety and activity of ivosidenib in patients with IDH1-mutant advanced cholangiocarcinoma: a phase 1 study. Lancet Gastroenterol Hepatol 2019;4:711-20.
24. Javle M, Borad MJ, Azad NS, et al. Pertuzumab and trastuzumab for HER2-positive, metastatic biliary tract cancer (MyPathway): a multicentre, open-label, phase 2a, multiple basket study. Lancet Oncol 2021;22:1290-300.
25. Harding J, Cleary J, Shapiro G, et al. Treating HER2-mutant advanced biliary tract cancer with neratinib: benefits of HER2-directed targeted therapy in the phase 2 SUMMIT ‘basket’ trial. Ann Oncol 2019;30:iv127.
26. Babina IS, Turner NC. Advances and challenges in targeting FGFR signalling in cancer. Nat Rev Cancer 2017;17:318-32.
27. Uson Junior PLS, Borad MJ. Precision approaches for cholangiocarcinoma: progress in clinical trials and beyond. Expert Opin Investig Drugs 2022;31:125-31.
28. Abou-Alfa GK, Sahai V, Hollebecque A, et al. Pemigatinib for previously treated, locally advanced or metastatic cholangiocarcinoma: a multicentre, open-label, phase 2 study. Lancet Oncol 2020;21:671-84.
29. Shi GM, Huang XY, Wen TF, et al. Pemigatinib in previously treated Chinese patients with locally advanced or metastatic cholangiocarcinoma carrying FGFR2 fusions or rearrangements: a phase II study. Cancer Med 2023;12:4137-46.
30. Bekaii-Saab TS, Valle JW, Van Cutsem E, et al. FIGHT-302: first-line pemigatinib vs gemcitabine plus cisplatin for advanced cholangiocarcinoma with FGFR2 rearrangements. Future Oncol 2020;16:2385-99.
31. Javle M, Lowery M, Shroff RT, et al. Phase II study of BGJ398 in patients with FGFR-altered advanced cholangiocarcinoma. J Clin Oncol 2018;36:276-82.
32. Javle M, Roychowdhury S, Kelley RK, et al. Infigratinib (BGJ398) in previously treated patients with advanced or metastatic cholangiocarcinoma with FGFR2 fusions or rearrangements: mature results from a multicentre, open-label, single-arm, phase 2 study. Lancet Gastroenterol 2021;6:803-15.
33. Makawita S, K Abou Alfa G, Roychowdhury S, et al. Infigratinib in patients with advanced cholangiocarcinoma with FGFR2 gene fusions/translocations: the PROOF 301 trial. Future Oncol 2020;16:2375-84.
34. Bahleda R, Meric-Bernstam F, Goyal L, et al. Phase I, first-in-human study of futibatinib, a highly selective, irreversible FGFR1-4 inhibitor in patients with advanced solid tumors. Ann Oncol 2020;31:1405-12.
35. Goyal L, Meric-Bernstam F, Hollebecque A, et al. Updated results of the FOENIX-CCA2 trial: efficacy and safety of futibatinib in intrahepatic cholangiocarcinoma (iCCA) harboring FGFR2 fusions/rearrangements. J Clin Oncol 2022;40:4009.
36. Borad MJ, Bridgewater JA, Morizane C, et al. A phase III study of futibatinib (TAS-120) versus gemcitabine-cisplatin (gem-cis) chemotherapy as first-line (1L) treatment for patients (pts) with advanced (adv) cholangiocarcinoma (CCA) harboring fibroblast growth factor receptor 2 ( FGFR2 ) gene rearrangements (FOENIX-CCA3). JCO 2020;38:TPS600.
37. Rizzo A, Ricci AD, Brandi G. IDH inhibitors in advanced cholangiocarcinoma: Another arrow in the quiver? Cancer Treat Res Commun 2021;27:100356.
38. Farshidfar F, Zheng S, Gingras MC, et al. Cancer Genome Atlas Network. Integrative genomic analysis of cholangiocarcinoma identifies distinct idh-mutant molecular profiles. Cell Rep 2017;18:2780-94.
39. Xiang X, Liu Z, Zhang C, et al. IDH mutation subgroup status associates with intratumor heterogeneity and the tumor microenvironment in intrahepatic cholangiocarcinoma. Adv Sci 2021;8:e2101230.
40. Lavacchi D, Caliman E, Rossi G, et al. Ivosidenib in IDH1-mutated cholangiocarcinoma: clinical evaluation and future directions. Pharmacol Ther 2022;237:108170.
41. Zhu AX, Macarulla T, Javle MM, et al. Final overall survival efficacy results of ivosidenib for patients with advanced cholangiocarcinoma with IDH1 mutation: the phase 3 randomized clinical ClarIDHy trial. JAMA Oncol 2021;7:1669-77.
42. Javle M, Bekaii-Saab T, Jain A, et al. Biliary cancer: utility of next-generation sequencing for clinical management. Cancer 2016;122:3838-47.
43. Li M, Zhang Z, Li X, et al. Whole-exome and targeted gene sequencing of gallbladder carcinoma identifies recurrent mutations in the ErbB pathway. Nat Genet 2014;46:872-6.
44. Ramanathan RK, Belani CP, Singh DA, et al. A phase II study of lapatinib in patients with advanced biliary tree and hepatocellular cancer. Cancer Chemother Pharmacol 2009;64:777-83.
45. Peck J, Wei L, Zalupski M, O'Neil B, Villalona Calero M, Bekaii-Saab T. HER2/neu may not be an interesting target in biliary cancers: results of an early phase II study with lapatinib. Oncology 2012;82:175-9.
46. Meric-Bernstam F, Beeram M, Hamilton E, et al. Zanidatamab, a novel bispecific antibody, for the treatment of locally advanced or metastatic HER2-expressing or HER2-amplified cancers: a phase 1, dose-escalation and expansion study. Lancet Oncol 2022;23:1558-70.
47. Ohba A, Morizane C, Ueno M, et al. Multicenter phase II trial of trastuzumab deruxtecan for HER2-positive unresectable or recurrent biliary tract cancer: HERB trial. Future Oncol 2022;18:2351-60.
48. Lee CK, Chon HJ, Cheon J, et al. Trastuzumab plus FOLFOX for HER2-positive biliary tract cancer refractory to gemcitabine and cisplatin: a multi-institutional phase 2 trial of the Korean cancer study group (KCSG-HB19-14). Lancet Gastroenterol 2023;8:56-65.
49. Xin HY, Sun RQ, Zou JX, et al. Association of BRAF variants with disease characteristics, prognosis, and targeted therapy response in intrahepatic cholangiocarcinoma. JAMA Netw Open 2023;6:e231476.
50. Subbiah V, Lassen U, Élez E, et al. Dabrafenib plus trametinib in patients with BRAFV600E-mutated biliary tract cancer (ROAR): a phase 2, open-label, single-arm, multicentre basket trial. Lancet Oncol 2020;21:1234-43.
51. Drilon A, Laetsch TW, Kummar S, et al. Efficacy of larotrectinib in TRK fusion-positive cancers in adults and children. N Engl J Med 2018;378:731-9.
52. Doebele RC, Drilon A, Paz-Ares L, et al. trial investigators. Entrectinib in patients with advanced or metastatic NTRK fusion-positive solid tumours: integrated analysis of three phase 1-2 trials. Lancet Oncol 2020;21:271-82.
53. Jakubowski CD, Azad NS. Immune checkpoint inhibitor therapy in biliary tract cancer (cholangiocarcinoma). Chin Clin Oncol 2020;9:2.
54. Fiste O, Ntanasis-Stathopoulos I, Gavriatopoulou M, et al. The emerging role of immunotherapy in intrahepatic cholangiocarcinoma. Vaccines 2021;9:422.
55. Jänne PA, Riely GJ, Gadgeel SM, et al. Adagrasib in non-small-cell lung cancer harboring a KRASG12C mutation. N Engl J Med 2022;387:120-31.
56. Awad MM, Liu S, Rybkin II, et al. Acquired resistance to KRASG12C Inhibition in cancer. N Engl J Med 2021;384:2382-93.
57. Sun J, Chen Q, Ma J. Notch-Sox9 axis mediates hepatocyte dedifferentiation in kras(G12V)-induced zebrafish hepatocellular carcinoma. Int J Mol Sci 2022;23:4705.
58. Zhou SL, Xin HY, Sun RQ, et al. Association of KRAS variant subtypes with survival and recurrence in patients with surgically treated intrahepatic cholangiocarcinoma. JAMA Surg 2022;157:59-65.
59. Cox AD, Fesik SW, Kimmelman AC, Luo J, Der CJ. Drugging the undruggable RAS: mission possible? Nat Rev Drug Discov 2014;13:828-51.
60. Pylayeva-Gupta Y, Grabocka E, Bar-Sagi D. RAS oncogenes: weaving a tumorigenic web. Nat Rev Cancer 2011;11:761-74.
62. Skoulidis F, Li BT, Dy GK, et al. Sotorasib for lung cancers with KRAS p.G12C mutation. N Engl J Med 2021;384:2371-81.
63. Sharma P, Hu-Lieskovan S, Wargo JA, Ribas A. Primary, adaptive, and acquired resistance to cancer immunotherapy. Cell 2017;168:707-23.
64. Berchuck JE, Facchinetti F, DiToro DF, et al. The clinical landscape of cell-free DNA alterations in 1671 patients with advanced biliary tract cancer. Ann Oncol 2022;33:1269-83.
65. Uson Junior PLS, Majeed U, Yin J, et al. Cell-free tumor dna dominant clone allele frequency is associated with poor outcomes in advanced biliary cancers treated with platinum-based chemotherapy. JCO Precis Oncol 2022;6:e2100274.
66. Goyal L, Saha SK, Liu LY, et al. Polyclonal secondary FGFR2 mutations drive acquired resistance to FGFR inhibition in patients with FGFR2 fusion-positive cholangiocarcinoma. Cancer Discov 2017;7:252-63.
67. Silverman IM, Hollebecque A, Friboulet L, et al. Clinicogenomic analysis of FGFR2-rearranged cholangiocarcinoma identifies correlates of response and mechanisms of resistance to pemigatinib. Cancer Discov 2021;11:326-39.
68. Lau DK, Jenkins L, Weickhardt A. Mechanisms of acquired resistance to fibroblast growth factor receptor targeted therapy. Cancer Drug Resist 2019;2:568-79.
69. Lavacchi D, Pellegrini E, Palmieri VE, et al. Immune checkpoint inhibitors in the treatment of renal cancer: current state and future perspective. Int J Mol Sci 2020;21:4691.
70. Feng K, Liu Y, Zhao Y, et al. Efficacy and biomarker analysis of nivolumab plus gemcitabine and cisplatin in patients with unresectable or metastatic biliary tract cancers: results from a phase II study. J Immunother Cancer 2020;8:e000367.
71. Mohan A, Griffith KA, Wuchu F, et al. Devimistat in combination with gemcitabine and cisplatin in biliary tract cancer: preclinical evaluation and phase Ib multicenter clinical trial (BilT-04). Clin Cancer Res 2023;29:2394-400.
72. Zeng TM, Yang G, Lou C, et al. Clinical and biomarker analyses of sintilimab plus gemcitabine and cisplatin as first-line treatment for patients with advanced biliary tract cancer. Nat Commun 2023;14:1340.
73. Lee HS, Han DH, Cho K, et al. Integrative analysis of multiple genomic data from intrahepatic cholangiocarcinoma organoids enables tumor subtyping. Nat Commun 2023;14:237.
74. Bao X, Li Q, Chen J, et al. Molecular subgroups of intrahepatic cholangiocarcinoma discovered by single-cell RNA sequencing-assisted multiomics analysis. Cancer Immunol Res 2022;10:811-28.
Cite This Article
Export citation file: BibTeX | RIS
OAE Style
Pan Sy, Ye YH, Zhou ZJ, Fan J, Zhou J, Zhou SL. Mutation-based therapies for intrahepatic cholangiocarcinoma: new options on the horizon. Hepatoma Res 2024;10:3. http://dx.doi.org/10.20517/2394-5079.2023.44
AMA Style
Pan Sy, Ye YH, Zhou ZJ, Fan J, Zhou J, Zhou SL. Mutation-based therapies for intrahepatic cholangiocarcinoma: new options on the horizon. Hepatoma Research. 2024; 10: 3. http://dx.doi.org/10.20517/2394-5079.2023.44
Chicago/Turabian Style
Pan, Si-yuan, Yu-Hang Ye, Zheng-Jun Zhou, Jia Fan, Jian Zhou, Shao-Lai Zhou. 2024. "Mutation-based therapies for intrahepatic cholangiocarcinoma: new options on the horizon" Hepatoma Research. 10: 3. http://dx.doi.org/10.20517/2394-5079.2023.44
ACS Style
Pan, S.y.; Ye Y.H.; Zhou Z.J.; Fan J.; Zhou J.; Zhou S.L. Mutation-based therapies for intrahepatic cholangiocarcinoma: new options on the horizon. Hepatoma. Res. 2024, 10, 3. http://dx.doi.org/10.20517/2394-5079.2023.44
About This Article
Special Issue
Copyright
Data & Comments
Data
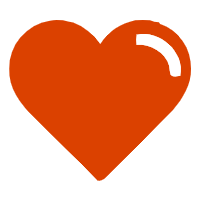

Comments
Comments must be written in English. Spam, offensive content, impersonation, and private information will not be permitted. If any comment is reported and identified as inappropriate content by OAE staff, the comment will be removed without notice. If you have any queries or need any help, please contact us at support@oaepublish.com.