MRI for screening and surveillance for hepatocellular cancer in NAFLD and NASH
Abstract
Chronic liver disease is an important risk factor for the development of hepatocellular carcinoma (HCC), with 80%-90% developing in the background of cirrhosis, primarily due to alcohol abuse and chronic viral infection with hepatitis B and C. Non-alcoholic fatty liver disease (NAFLD) and non-alcoholic steatohepatitis (NASH) related HCC is rising due to the global epidemic of obesity and diabetes. NAFLD also potentiates other risk factors of HCC, such as chronic hepatitis C and alcoholic liver injury. Furthermore, HCC may complicate non-cirrhotic NAFLD, greatly expanding the population potentially at risk. Screening and surveillance for HCC with ultrasonography (US) in this patient population pose challenges related to body habitus, liver morphology, and co-morbidities. Magnetic resonance imaging (MRI) applying various image sequence protocols and contrast agents could aid in early detection and improved prognosis of HCC. It is of utmost importance to define the most cost-effective dynamic imaging protocol for use in patients where high-quality ultrasonography images cannot be obtained and for patients undergoing surveillance for lesions identified with US. Furthermore, standardization of MRI protocols will help to define potential unique HCC features in this population. A scoring system including patient-derived factors may help to identify high-risk patients. Standardized protocols as part of prospective cohort studies and randomized clinical trials will help to stratify high-risk patients and to aid the development of professional guidelines for screening and surveillance of HCC in patients with NAFLD and NASH using dynamic imagining techniques.
Keywords
INTRODUCTION
Chronic liver disease (CLD) poses the most significant risk for the development of hepatocellular carcinoma (HCC), with 80%-90% of cases developing in the background of cirrhosis, primarily due to chronic viral infection with hepatitis B and C, alcohol misuse, and metabolic disorders such as hemochromatosis. NAFLD (non-alcoholic fatty liver disease) and NASH (non-alcoholic steatohepatitis) are the hepatic manifestations of metabolic syndrome, increasingly leading to CLD, HCC, liver-related mortality, and liver transplantation. Over the next decade, NASH and related complications are expected to emerge as the leading cause of liver transplantation in the United States[1].
Furthermore, complications of NAFLD-related liver disease often progress slowly and silently, which makes it difficult to identify at-risk patients and complicates introductions of HCC screening programs.
Epidemiological cohort data support surveillance of patients with NAFLD based on the incidence of HCC. The fact that HCC can occur in NAFLD in the absence of cirrhosis raises the question of whether and when to consider screening for these patients with advanced fibrosis. A recent expert review from the American Gastroenterology Association (AGA) recommends considering HCC surveillance in patients with NAFLD-related cirrhosis or advanced fibrosis if non-invasive tests such as shear wave elastography, MR elastography, or laboratory scores including fibrosis-4 (FIB-4) index, or the enhanced liver fibrosis (ELF) test indicate significant fibrosis[2].
Among patients with NASH cirrhosis, the annual incidence of HCC ranges from 0.5%-2.6%, whereas the incidence of HCC in non-cirrhotic NAFLD is much lower at 0.1-1.3 per 1,000 patient-years[3]. Several studies report that NAFLD patients lacking background cirrhosis had a significantly larger tumor burden at the time of diagnosis compared to patients with established cirrhosis pointing to a poorer prognosis[4,5]. It remains to be speculated if the larger tumor burden and diagnosis of advanced HCC may be attributed to less screening in NAFLD patients, especially those without established cirrhosis. Another study shows that the rate of microscopic vascular invasion is significantly higher in NAFLD patients compared to other etiologies (65% vs. 30%; P = 0.027), suggesting that NAFLD may support a permissive microenvironment for HCC progression[6]. A large cross-sectional study on an inpatient sample of 30,712,524 hospitalizations of adult patients reports a higher HCC prevalence of 0.50% (95%CI: 0.41-0.59) in patients with NASH compared to 0.21% (95%CI: 0.20-0.23) in patients without NASH (P < 0.001). Patients with NASH are about 60% more likely to have HCC compared to those without NASH (adjusted OR: 1.6, 95%CI:1.4-1.9, P < 0.001), even after adjustments for age, gender, obesity, alcohol use, tobacco use, hepatitis B and C, HIV, hemochromatosis, background cirrhosis as well as other co-morbidities were made[7]. Patients with metabolic syndrome and HCC appear to be older (mean age: 67 vs. 59 years, P < 0.01), although the background liver shows significantly lower fibrosis stage 0-2 (65%) compared to patients with other etiologies (26%; P < 0.001)[6].
Dynamic imaging using MRI plays a steadily increasing and important role in patients with CLD. Recent advances in technology, such as perfusion-weighted MRI, diffusion-weighted MRI, MR elastography, and MR spectroscopy, allow a non-invasive, multi-faceted, and accurate assessment of the patients' hepatic anatomy, hepatic composition, stage of fibrosis, and the detection and characterization of hepatic lesions including HCC.
HCC has high rates of death and recurrence and, overall, a very low survival rate. Novel systemic therapies, particularly immune checkpoint inhibitors, have emerged as monotherapy or in combination with various chemotherapy agents[8]. Most recently, a combination of atezolizumab and bevacizumab was accepted as the first-line treatment for advanced HCC[9,10]. Durvalumab plus tremelimumab, atezolizumab plus cabozantinib, pembrolizumab monotherapy, and the combination of nivolumab plus ipilimumab - all have provided superior overall survival or superior progression-free survival. These regimens received FDA accelerated approval as second-line treatment based on early efficacy data. Despite the impressive results and overall acceptable side effect profile of these drugs, only a subset of patients will respond to therapy. Biomarker-based stratification of patients, who may respond to immunotherapy, is an unmet need. Genomic profiling to analyze gene alterations to determine the tumor mutational burden (TMB), microsatellite instability, and mismatch repair deficiencies may aid in identifying meaningful predictive biomarkers[9,11]. Dynamic imaging identifies early treatment responses and helps to guide further therapy. Emerging data suggest that MRI radiomic features could be considered as a potential “imaging” biomarker to assess the histological grade, status of microvascular invasion, the status of gene expression, and response to local and systemic therapy[12]. There is also increasing evidence that gadolinium-enhanced dynamic liver MRI “phenotypes” correlate with specific genetic, molecular, and histologic characteristics of different HCC subtypes[13]. This article provides an overview of the role of MRI in screening and surveillance for HCC in patients with NAFLD and NASH.
WHEN AND HOW SHOULD WE SCREEN FOR HCC IN NAFLD/NASH?
There is consensus among professional guidelines, including the American Association for the Study of Liver Disease (AASLD) and the European Association for the Study of the Liver (EASL), recommending surveillance for HCC in patients with cirrhosis based on clinical context and indicators of advanced liver disease with or without biopsy confirmation[14,15]. Screening should be performed with ultrasonography (US) with or without the addition of alphafetoprotein (AFP).
How to identify patients with cirrhosis?
To date, there is not enough evidence to support surveillance for patients with NAFLD without cirrhosis, although HCC due to non-cirrhotic NASH is a rising concern. Establishing the diagnosis of cirrhosis in these patients has its challenges. In clinical practice, we often use non-invasive tests for the assessment of liver fibrosis to risk-stratify these patients. Cost-effective appears point-of-care testing by combining patient demographics and laboratory tests, such as the aspartate aminotransferase (AST) to alanine aminotransferase (ALT) ratio, AST to platelet ratio index (APRI), the NAFLD fibrosis score (age, BMI, AST, ALT, platelets, albumin, diabetes/impaired fasting glucose), and FIB-4 score (age, AST, ALT, platelets). A FIB-4 score greater than 2.67 is associated with higher odds of having cirrhosis or bridging fibrosis[16]. Nonetheless, a significant limitation of all these markers is the high false negative rate in the detection of significant fibrosis, which has been reported to be up to 40%[16]. Results from transient elastography (TE), ultrasound shear wave elastography (SWE), and MR elastography (MRE) may help establish the diagnosis of bridging fibrosis or cirrhosis but must be incorporated with clinical data.
Liver fibrosis progression does not follow a linear course. Regression can occur up to the stage of bridging fibrosis (F3). Many factors, including older age, obesity, insulin resistance/diabetes, hypertension, and certain variants in PNPLA3, MBOAT7, TM6SF2, and HSD13B17 genes[17], can promote fibrosis. The stage of fibrosis is the main driver for the development of liver decompensation and HCC. Identifying high-risk groups for potentially more rapid fibrosis progression is crucial. The EASL guidelines recommend specifically evaluating patients with obesity and diabetes as those have a 2 to 3-fold risk of developing ESLD and HCC[18].
What are the differences in performance and cost between ultrasonography and MRI?
As a curative treatment, including liver transplantation and with prognosis related to tumor size, the goal of screening and surveillance is to identify small HCCs amenable to curative treatment. Once patients with NAFLD are considered to be at high risk for HCC, they often prove to be poor candidates for ultrasonography, given their body habitus and abdominal obesity. Indirect signs, such as nodular liver surface, splenomegaly, and intra-abdominal varices, may help to establish the diagnosis of cirrhosis. US is cost-effective but has low sensitivity in obese individuals with fatty liver. The attenuation of images quickly deteriorates within 4-5 cm of depth, making deeper structures difficult to evaluate[19]. A negative US in the context of an ultrasound visualization score of C is associated with reduced sensitivity in detecting HCC. Furthermore, the performance of US in early HCC detection, even with high-quality equipment, is highly operator-dependent.
The quantification of liver fat by ultrasonography lacks a metric, which is reproducible and matches the accuracy of MRI techniques, such as proton density fat fraction (PDFF) or MR spectroscopy. Currently, B-mode US is the most available technique, which only provides a qualitative assessment of the degree of steatosis[20]. Current techniques provide an indirect estimate of liver fat and are subject to inter-observer variability, making sequential comparison somewhat unreliable. The performance of the B-mode technique is closer to the MRI technique at levels of liver fat content of at least 20%-30%, but more inconsistent at lower levels of liver fat content. US for quantitative liver fat assessment is based on US beam attenuation i.e., Fibroscan, which provides acceptable performance in the overall grading of liver steatosis. The dilemma is that this technique lacks correct identification of the amount of liver fat around the cut-off points for the degree of steatosis. There are some emerging calculators for fat content measurement using ultrasonography, which are comparable to the MRI PDFF estimations. At present, these lack consistency across vendors, are experimental, and are not available for routine clinical use[21].
Currently, MRI-derived PDFF measurements are reliable, consistent, and correlate well with biopsy results at all levels of liver fat content[20].
The costs of MRI for HCC screening are currently being addressed by emerging focused or abbreviated studies, at least in the United States[21]. A limited non-contrast charge for abdominal MRI has a reimbursement, which is comparable to the reimbursement for complete ultrasonography of the abdomen. The duration of the examination is about 10-15 minutes, which further enhances patient compliance. Unlike US, it is reproducible and has a more consistent performance.
For surveillance in general, both multiphase CT and dynamic MRI are not cost-effective, particularly due to a considerable rate of false-positive imaging findings, which require further workup. However, for patients listed for liver transplantation, multiphase CT or MRI are suitable alternatives to US. As US is particularly insensitive to small lesions less than 1 cm in size, it can lead to surveillance failure resulting in a more advanced tumor stage at initial presentation. Any newly developed hepatic mass of 1 cm or greater in size without typical findings of a simple cyst or typical hemangioma should be regarded as suspicious for HCC. A prospective study comparing visualization scores of US compared to MRI showed that one-third of patients with NAFLD-related cirrhosis had significantly limited visualization[22].
Following the current guidelines, we may miss HCC in patients with NAFLD/NASH who are not labeled “cirrhotic”, yet, and therefore not offered regular US exams. Using non-invasive laboratory and imaging tests will help to identify patients with advanced fibrosis and cirrhosis to connect them to an HCC screening program. Furthermore, small lesions suitable for potentially curative treatment are being missed in US due to poor visibility related to the patient's body habitus and liver steatosis. High-quality equipment and skilled operators are of utmost importance in the use of ultrasonography for HCC screening [Figure 1].
What about contrast-enhanced ultrasonography?
Contrast-enhanced ultrasound (CEUS) for the characterization of focal liver lesions has been used in Europe and Asia for decades. The United States Food and Drug Administration approved the contrast agent Lumason® (sulfur hexafluoride microbubbles) in 2014. Nonetheless, CEUS is not widely accepted in the U.S.. Initially, the AASLD included CEUS in the diagnostic algorithm for liver lesions, along with CT and MRI. In 2010, the CEUS was removed from the guidelines due to the potential of misdiagnosing intra-hepatic cholangiocarcinoma (ICCA) as HCC. The most recent AASLD guidelines from 2018 still do not include CEUS as a recommended diagnostic modality[14]. The recommendation is based on a meta-analysis from Asia which reported encouraging results with a sensitivity of 85% (95%CI: 84-85) and a specificity of 91% (95%CI: 90-92), respectively[23]. Unfortunately, this included only patients who would have an adequate quality US. Additional drawbacks were small cohort sizes, lack of generalizability, as well as publication bias[23]. Prospective studies in the U.S. and other Western cohorts are needed to verify these results. American hepatology and radiology societies disagree on whether CEUS should be incorporated in characterizing liver lesions. In 2017, the American College of Radiology (ACR) Liver Imaging Reporting and Data System (LI-RADS) updated its CEUS-LiRADS criteria to classify untreated liver observations with different likelihood levels in patients at high risk for HCC[24,25].
Recent studies show that MRI and CEUS perform similarly in diagnosing non-HCC malignancies[26] and in primary surveillance[27,28]. To monitor for HCC recurrence[29], CEUS and MRI show a similar relative diagnostic test accuracy. Moreover, CEUS can resolve equivocal results on MRI in situations when tumor recurrence cannot not be clearly distinguished from post-treatment changes or shunting. A systematic review, including cost-effectiveness analysis, confirms that CEUS provides similar diagnostic performance to contrast-enhanced dynamic CT or MRI for the assessment of focal liver lesions and was considered to be a cost-effective replacement for MRI and CT in the surveillance of patients with cirrhosis and the assessment of incidentally detected focal liver lesions[30].
SHOULD WE USE MRI FOR SCREENING/SURVEILLANCE FOR HCC?
General considerations
Abdominal computed tomography and magnetic resonance imaging are more sensitive for HCC detection compared to US; thus, they may have a role as an additional screening modality for early HCC detection to improve survival and prognosis[31,32]. However, those exams also have disadvantages. The use of CT is limited by exposure to radiation as well as the need for potential nephrotoxic contrast agents[33]. Contrast-enhanced MRI provides superior tissue contrast without the risk of radiation. It is considered an optimal modality for lesion detection and differential diagnosis by a growing number of clinicians[32-39]. Limiting factors include high cost, availability, and complexity of the exam leading to long examination times. There is also a risk of side effects related to the contrast agents, specifically gadolinium[40].
According to results of a large prospective multicenter French cohort study comparing US and MRI in 2513 patients with compensated cirrhosis, MRI detected 5 times more early-stage HCC (Barcelona Clinic Liver Cancer, BCLC 0) than US. The authors conclude that MRI is cost-effective for early HCC detection assuming an annual HCC risk of greater than 3%[41]. Subsequent studies have substantiated the efficacy of MRI as a screening and surveillance tool for HCC[34,42].
There is no consent and standardization of MRI protocols across institutions using different imaging sequences and contrast agents or no contrast at all. MAGNUS-HCC, a current, prospective, multicenter clinical trial in Korea is comparing US every 6 months and non-contrast MRI every 12 months for detection of HCC during a follow-up period of 3 years[43].
In general, optimal MRI sequences include T2-weighted imaging (T2WI) which is highly sensitive in detecting focal liver lesions, while sequences using diffusion-weighted imaging (DWI) appear to be more effective for the detection and characterization of HCC in cirrhotic livers[44], especially for small tumors less than 2 cm in size. High DWI b-values have proven to be helpful in distinguishing benign dysplastic nodules from HCC[45]. An exam with these basic MRI sequences, even without contrast agents, appears to be quite adequate for HCC screening as an alternative to US. Exam time is only 10-15 minutes, which is much shorter compared to a regular contrast-enhanced dynamic MRI.
MRI protocols for imaging HCC, the technicalities
Liver imaging with MRI requires a standardized imaging technique for effective follow-up of liver lesions, which is consistent, reproducible, and accurate. There are several societal guidelines in different regions of the world. The LI-RADS (Liver Reporting and Data System) has evolved as the leader in North America, aligning with the AASLD guidelines and reporting requirements of regulatory entities such as UNOS/OPTN (United Network for Organ Sharing/Organ Procurement and Transplantation Network) to provide consistency for reporting liver lesions. The technical considerations include 1.5 or 3.0 Tesla scanners utilizing a multichannel torso and phased array coil. The scanning protocol must utilize a multiphase 3D series and must include a non-contrast phase (pre-contrast). Following iv contrast administration of a gadolinium-based agent (GDBA), images are acquired in the late arterial phase, portal venous phase, and delayed phase at 2-5 min[46]. Using hepatobiliary (HBC) agents, the delayed phase is called the transitional phase, and an additional phase at 20 minutes post iv contrast injection is added (hepatobiliary phase). Furthermore, to improve image quality, the incorporation of fat suppression and subtraction images is desirable. Other optional sequences include pre-contrast in-and-out-of-phase images, T2-weighted images with fat suppression, as well as DWI images. The trade-off to improved quality is an increase in exam time.
Contrast injection specifications are dependent on the contrast agent. For ECA, dosing is weight-based at 0.1m mmol/kg body weight at an injection rate of 1-2 mL/sec, followed by a saline bolus 30-40 mL at
General indications for liver MRI based on appropriateness criteria of the American college of radiology
Screening and surveillance in patients with CLD without a prior diagnosis of HCC
The American College of Radiology (ACR) appropriateness criteria state that abdominal US and MRI abdomen with/without hepatobiliary contrast are usually appropriate in this patient population, while MRI abdomen without contrast may be appropriate. These specific recommendations are not supported by any of the hepatology societies, including AASLD, EASL, and the Asian Pacific Associations for the Study of the Liver (APASL), given the paucity of data on their efficacy and cost-effectiveness. However, there is more and more agreement that MRI may be beneficial in patients, who are at high risk, but difficult to image by ultrasound due to high BMI or abdominal obesity[48].
Detection and characterization of HCC less than 2 cm in diameter
MRI has a reported sensitivity between 47 and 95% in the detection of lesions less than 2cm. It is considered as an appropriate additional imaging modality in patients with nodules seen on US and particularly helpful in the characterization of small lesions less than 1cm in the background of CLD.
Monitoring patients with HCC undergoing loco-regional therapies including ablative therapies, SBRT, bland embolization, chemoembolization or radioembolization.
Follow-up MRI with/without contrast is usually appropriate and obtained at 1 month, and subsequent 3 months intervals up to 2 years from initial ablation. It serves as bridge surveillance to liver transplant[48].
Non-contrast quantitative MRI examinations
This emerging tool aids in determining the extent of the underlying CLD. These studies include MR elastography sequences to quantify liver stiffness and proton density fat fraction to determine the degree of hepatic steatosis, supporting the diagnosis of early-stage NAFLD, and to monitor therapy including lifestyle modification.
WHICH CONTRAST AGENT AND WHICH PROTOCOL IS BEST IN GENERAL AND FOR NAFLD PATIENTS IN PARTICULAR?
In general, MRI contrast agents detect and describe liver lesions based on blood flow, while hepatobiliary agents (HBA) provide additional information on hepatocellular appearance. In 2018, AASLD and EASL updated their guidelines for the diagnosis of HCC by including the adoption of HBA-MRI of the liver, in addition to the conventional extracellular contrast agent (ECA). The main advantage of ECA-MRI imaging lies in its high specificity for the diagnosis of HCC[49]. A higher sensitivity of ECA-MRI (77.9 vs. 66.3%) and accuracy (82.1 vs. 72.6%) compared to HBA-MRI for detecting LIRADS-5 lesions (P < 0.001) has been documented[50]. However, a recent meta-analysis shows similar sensitivity of ECA-MRI and HBA-MRI for the detection of HCC combined with a higher sensitivity of up to 96%[51]. Although to date, the ACR guidance document states that evidence to recommend one contrast agent type over another is insufficient[14]. Patients with NAFLD often have dense and cirrhotic livers in US, making the diagnosis of lesions challenging. Gadolinium-based contrast media are ideal for all NAFLD patients if MRI is added to screening protocols or used for surveillance for HCC.
Abbreviated protocols include 3 phases: a) a hepatobiliary phase (HBP) T1-weighted imaging sequence which detects the altered expression of the OATP1B3 transporter regulating gadoxetate uptake into hepatocytes[52]; b) a diffusion-weighted imaging (DWI) sequence with sensitivity on high cellularity independent of OATP1B3 transporter expression[53]; and c) a T2-weighted single fast spin-echo image sequence to separate cysts and hemangiomas[54].
Extracellular contrast agents
These agents arrive in the liver via the hepatic artery and portal vein. There is delayed transit through the interstitial (pre-sinusoidal) space without uptake by the hepatocytes, or excretion from the biliary tract. The kidneys exclusively excrete contrast. Prototypes of gadolinium-based extracellular agents include gadobutrol (GADAVIST©) and gadoterate meglumine (DOTAREM©)[Figure 2 A-D].
Hepatobiliary agents (intracellular)
These contrast agents arrive in the liver via the hepatic artery and portal vein with subsequent distribution into the interstitial space and additional uptake into the hepatocytes via the OATP receptors and finally excretion through the biliary tract. Gadoxetic acid (EOVIST©) is the prototype used in the United States, while gadoxetate disodium (PRIMOVIST©) is used in Europe. A well-documented advantage of using HBA includes better lesion visibility on 20 minutes-delayed images. Furthermore, some studies report that the peri-tumor extent and invasion can be better assessed and serves as an independent predictor for HCC recurrence after loco-regional treatment or resection[55-57]. In addition, satellite nodules and pseudo-lesions can be differentiated with higher accuracy[58]. The challenges of imaging a cirrhotic liver with an HBA still outweigh the benefit of delayed imaging in the hepatobiliary phase, although decreasing OATP receptors documented in cirrhosis prolong the study time. Timing of the hepatobiliary phase may be as long as 60 minutes compared to 20 minutes for patients with normal liver function. Optimal volume and speed of contrast media delivery are of utmost importance during the arterial phase to assure optimal arterial enhancement of lesions. Transient tachypnea can be seen at high injection rates leading to motion artifacts, often counteracted by injecting a larger dose at a slower injection rate which, however, is not optimal for the arterial phase bolus[47,59,60]. This approach reduces lesion discernibility in the arterial phase and leads to less washout. The transitional phase is not used for washout determination due to significant hepatocellular uptake of contrast 2 to 5 minutes after injection. A lesion capsule is less visible on the background of a brighter liver. Other factors, such as iron overload, additionally affect the degree of arterial enhancement. Hepatic steatosis may interfere with the degree of enhancement and is less noticeable on the T1-weighted images. Ideally, images should be scanned without fat suppression and using the in-phase echo time. However, these strategies deviate from standard protocols and are not widely implemented[48] [Figure 3 A-E].
Abbreviated MRI protocols
Different imaging strategies are currently being investigated. The ideal MRI protocol would provide a short imaging sequence detecting lesions less than 2.0 cm in size, in difficult-to-image livers, effectively and consistently, and is cost-effective. Overall, contrast-enhanced dynamic MRI with ECA using the dynamic contrast sequences as the focal sequence for HCC detection aligns well with most interpretation guidelines, including United Network for Organ Sharing (UNOS) and the Liver Imaging Reporting and Data System (LI-RADS)[61,62].
A second strategy involves HBA-MRI using key DWI-sequences and T2-weighted sequences, along with a post-contrast sequence in the hepatobiliary phase. This protocol utilizes the ancillary features of LI-RADS, such as non-enhancing capsules, mosaic architecture, nodule-in-nodule detection, or visibility of blood products or fat within the tumor[63].
A third option is to perform a non-contrast study, and supplement it with a contrast-enhanced MRI, if suspicious findings are identified. This protocol is more quantitative and includes sequences for liver stiffness calculations as well as quantification of iron and fat in the liver parenchyma[48], and may emerge as the preferred technique for screening patients with NAFLD/NASH in the future.
To date, there are no prospective controlled trials testing or comparing the above techniques. Those currently underway include the MIRACLE-HCC trial, which is evaluating the efficacy of non-contrast MRI compared to US every 6 months combined with AFP every 6 months[64]. The MAGNUS-HCC trial is underway in Korea, evaluating non-contrast MRI with ultrasonography at 12 months intervals[43]. In the U.S., FAST (Focused Abbreviated Screening Technique)-MRI, a study comparing an abbreviated MRI with ultrasonography for screening of HCC, is currently ongoing[65].
One analysis suggests that gadoxetate-enhanced aMRI offers substantial cost savings over conventional MRI. The authors report that the aMRI protocol was effective compared with semiannual US assuming any HCC prevalence. Dynamic aMRI and HBP-aMRI were found to be cost-effective strategies and associated with life-year gains of 12, 7, and 5 months for dynamic aMRI and 9, 6, and 4 months for HBP-aMRI with an HCC annual prevalence of 5.5%, 3%, and 2% when compared with US[66]. Another retrospective study by Chan et al. shows that a non-contrast aMRI had a per-patient negative predictive value (NPV) of 97% (95%CI: 95%-98%), which was not significantly different between patients with steatosis with an NPV of 99% (95%CI: 93%-100%), and without steatosis with an NPV of 97% (95%CI: 94%-98%). The reported per-patient sensitivity and specificity were 85% (95%CI: 75%-91%) and 93% (95%CI: 90%-95%), respectively[67]. The authors conclude that this non-contrast aMRI for HCC screening was unaffected by hepatic steatosis, and is highly specific with a sensitivity, well comparable to US and HBA-MRI. Specific patient characteristics, such as lean NAFLD, or concurrent other etiologies, such as viral hepatitis in an Asian population, might be the reason for these findings.
PROS AND CONS OF USING MRI FOR HCC SCREENING IN NAFLD
Advantages of imaging NAFLD-related HCC with MRI
Current studies evaluating the efficacy of MRI compared to US are mostly retrospective, with limited data analysis extracted from complete examinations. Data show a sensitivity of 85%-92% and specificity of 89%-100% for MRI with ECA[68]. Similar retrospective studies of MRI with HBP agents show a sensitivity of 81%-92% with an NPV of 90-96%. Historical data report a significantly lower sensitivity for screening ultrasound at 47%-63%. Screening with multiphase CT on an annual basis has a sensitivity of 68%-73% for detecting HCC[69]. When observations are imaged, both by CT and MRI, 49% are seen only on MRI and 2% are visible only on CT, suggesting MRI has a superior sensitivity compared to CT[37]. In addition, MRI screening exams could be combined with fat quantification and MR elastography to provide further risk assessment and stratification of high-risk patients [Figure 4 A-E].
Figure 4 A-E. uantitative non-contrast Amri. Vibration image of MRE; Color map of MRE; Grey-scale elastogram showing normal liver stiffness; PDFF image, Fat content in right hepatic lobe at 5%; T2* image for liver iron in the normal range.
According to a study on 21 patients with NASH-HCC conducted by Al-Sharhan et al.[5], 57% of NASH-HCC were less than 2 cm in size, and 40% did not display typical washout on MRI, questioning if classical diagnostic imaging criteria can be applied to NASH-HCC.
Patients with NAFLD/NASH with severe hepatic steatosis and fibrosis are difficult to image by US, the recommended screening modality by most clinical practice guidelines worldwide. Clinical trials to further study the role of aMRI are urgently needed. Cases of NAFLD-related HCC are rising and there is an alarming proportion of cases reported, up to 46% with NAFLD-associated liver disease, who develop malignancy without cirrhosis[3]. In the US, lesions are frequently referred for loco-regional therapy without biopsy, making LI-RADS criteria reproducibility important.
Disadvantages of MR imaging
MRI imaging is contraindicated in patients with pacemakers, which are non-conditional. Previously placed aneurysm clips, embolization coils, or stimulators, among other devices, may have MRI safety issues. The presence of ascites often limits the quality of images due to movement artifacts. It is preferable to perform paracentesis for large-volume ascites prior to MRI scanning. Repeated MRI examinations decrease the cost-effectiveness of surveillance in all except for patients on the liver transplant waiting list.
MRI DIAGNOSTIC CRITERIA FOR HCC
Defining HCC
For defining diagnostic criteria for HCC by MRI in North America, LI-RADS, under collaboration with the American College of Radiology[48], has been accepted as the guidance document. Over the last decade, ACR guidance has been integrated with the recommendations from AASLD and is now aligned with organizations regulating liver transplants (UNOS/OPTN). It is important to assure consistency in the interpretations, which has resulted in decreasing the need for biopsy of detected lesions. The consistency in reporting allows effective communication between radiologists as well as with referring physicians[9].
Findings are called “observation" or nodules if less than 2cm in size and distinct from the background. Larger observations, often called "masses", lead to displacement or compression of liver parenchyma. They may have an expansile, retractile, or diffuse appearance, and may deform the liver surface, either with well-circumscribed or not well-defined margins. A detailed discussion of all LI-RADS categories is beyond the scope of this paper; however, it should be pointed out that essential elements of the LI-RADS 5 lesion, confirming "definite HCC" are found in the post-contrast image series. The key components include non-rim enhancement on the delayed arterial phase, washout on portal venous or delayed phase[70], and/or an enhancing capsule. If the patient has previous studies within the last 6 months, threshold growth (TG) can be assessed as growth in the largest dimension of greater than 50% over a period of fewer than 6 months. Overall, retrospective data comparing the LI-RADS criteria in patients with HCC due to NASH or virus-induced chronic liver disease shows no differences in major criteria[71].
So-called ancillary findings cannot upgrade an observation to the LI-RADS 5 category but can aid in the diagnostic approach in patients under HCC surveillance. The findings include discrete nodules visible on US, sub-threshold growth, mild to moderate T2 hyper-intensity, restricted diffusion, corona enhancement, hepatobiliary phase hypo-intensity, as well as fat or iron sparing in a solid mass[62]. Importantly, the classification of lesions using LI-RADS applies only to patients with cirrhosis. To date, it remains largely unclear whether and how the LI-RADS criteria can be applied to HCC in NAFLD/NASH without cirrhosis. Further studies are urgently needed to establish diagnostic imaging criteria and protocols to serve this growing subset of individuals now developing HCC[72].
A recent study from Italy reports on patients with HCC but without cirrhosis and persistently normal transaminases, who were mostly older males with a history of type 2 diabetes. The criteria for screening and surveillance in these subsets of patients are not well established[73]. Clinically, patients tend to present later with poorer long-term survival. To date, data show that the imaging criteria of HCC in those patients are similar. Published data on specific imaging findings of HCC in NAFL/NASH are insufficient and summarized in Table 1. Unfortunately, there are no large studies to distinguish imaging features of NAFLD/NASH-related HCC from standard diagnostic imaging criteria.
HCC related to NAFLD/NASH
Patient Population | HCC Detailed Characteristics | Conclusion | |
Al-Sharhan et al.[5] | 21 pts. with NASH | Single HCC: 17/30 (57%) with size < 20 mm. Signal drop between in- and out-of-phase T1-weighted images: 16/30 HCC (53%). APHE: all HCC (30/30, 100%) No washout:12/30 HCC (40%) Encapsulation: 18/30 HCC (60%) Cirrhosis: 16/21 (76%) | 57% of NASH-HCC are < 2 cm, and 40% lack washout Classical imaging criteria should be applied with caution to HCC in NASH |
Yamaoka et al.[92] | Retrospective cohort of 535 pts with HCC from 1/2015-12/2020 Compared typical NASH-HCC (T-NASH-HCC) vs. partial-NASH-HCC (P-NASH-HCC) vs. other etiologies (O-HCC) | T-NASH-HCC: 11% of all HCC; 58 (97%) solitary, 2 (3%) multifocal P-NASH-HCC: 40 (89%) solitary, 5 (11%) multifocal T-NASH-HCC: smaller than O-NASH-HCC (P = 0.011) with higher background steatosis (P < 0.001) F4-fibrosis: 44% of T-NASH-HCC vs. 44% of P-NASH-HCC vs. 31% of O-HCC Diagnostic imaging: T-NASH-HCC vs. P-NASH-HCC vs. O-HCC: 87% of NASH-HCC were LR-5 | NASH-HCC can be diagnosed by combining US, CT, and MRI Diagnostic accuracy 93% (95%CI: 0.946-0.987), sensitivity 78.9%, specificity 99.9%, PPV 96.8%, NPV 97.2% |
Barat et al.[71] | Comparison of MRIs of 41 HCC in NASH pts. to 41 HCC in virus-induced CLD | No significant differences between NASH-HCC vs. virus-HCC Most common: arterial hyperenhancement and visibility on T2WI (93% vs. 98%, P = 0.616 and 85% vs. 88%, P = 1.000) No differences for any major LI-RADS v2018 criteria Strong inter-reader agreement for LI-RADS in NASH-HCC (kappa = 0.802); moderate in virus-HCC (kappa = 0.720) | The LI-RADS v2018 diagnostic algorithm can be applied to patients with NASH. |
Park et al.[93] | MEDLINE, EMBASE, Cochrane Library Search for studies 1/1/11 -7/19/21 on imaging features of HCC in NAFLD vs. NASH 5 studies (170 pts with 193 HCC) were included in the analysis | Pooled percentages of APHE, washout, and enhancing capsule were 94.0% (95%CI: 89.1-96.7%), 72.7% (95%CI: 63.3-80.4%), and 57.5% (95%CI: 45.1-69.1%), respectively with no significant difference between NAFLD and NASH (P ≥ 0.21). MRI and CT had a similar pooled percentage of APHE (94.3% vs. 93.4%, P = 0.82) and washout (70.4% vs. 77.2%, P = 0.38), but a higher pooled % of enhancing capsule (67.1% vs. 44.7%, P = 0.02) | HCC in NAFLD and NASH had a similar frequency of APHE compared to other etiology HCC but a relatively low frequency of washout and enhancing capsule |
Thompson et al.[72] | MRI review of 48 consecutive pts with NAFLD and biopsy-proven HCC (mean ± SD; 4.5 ± 3.4 cm) by 3 radiologists | Major HCC features APHE in 45 (93%), PVWO in 30 (63%), DPWO in 38 (79%), and enhancing "capsule" in 34 (71%). Cirrhotic morphology in 22 (46%). Inter-rater agreement: 0.75 for APHE, 0.42-0.58 for PVWO, 0.58-0.71 for DPWO, 0.38-0.67 for enhancing "capsule". 18%, 14%, and 22% increase in the odds of lack of PVWO, DPWO, and capsule for every 1% increase in hepatic steatosis without cirrhosis (P = 0.011, 0.040, and 0.029, respectively) | Absent washout and capsule are associated with increasing hepatic steatosis in pts with non-cirrhotic, NAFLD-associated HCC |
Effect of Steatosis on Diagnostic Criteria
A study on 48 cases with NAFLD from the Mayo Clinic[72] reports on the presence of washout, the presence of an enhancing capsule, and the amount of fat content in focal hepatic masses. There was a direct correlation between higher fat content and the absence of delayed washout, lack of capsule visibility, and absence of cirrhosis. The study reports that 56% of HCC occurred in livers with a non-cirrhotic morphology.
Digital subtraction post-processing may help to improve the visibility of washout and capsule. Some minor criteria, such as T2-hyperintensity and DWI-hyperintensity, appear to be more common in non-cirrhotic NAFLD. Future use of proton density fat fraction (PDFF) for fat quantification may be of value but is not a part of standard imaging protocols.
Simple hepatic steatosis, NAFLD, and NASH certainly pose different levels of risk in promoting and advancing the development of HCC. A meta-analysis of 19 studies showed that NASH was associated with a 2.6-fold higher risk of developing HCC in non-cirrhotic patients[73] The risk of HCC was increased by 5.5-8.6-fold in patients with concomitant diabetes and other features of metabolic syndrome[73]. Subsequently, this may represent a cohort of patients who could potentially benefit from integrating MRI into HCC screening and surveillance programs.
OUTLOOK FOR THE FUTURE [Figure 5]
Optimizing imaging tests
The use of MRI in patients with NAFLD and NASH continues to evolve. So far, available data are limited and inconsistent. To date, there are no professional practice guidelines recommending routine use of MRI for HCC screening or surveillance, in general, and specifically in patients with NAFLD/NASH based on lack of evidence and cost-effectiveness.
However, the utility of MRI goes well beyond tumor detection. It certainly can provide an additional assessment of fat content and stage of fibrosis, helping to identify high-risk patients. The utility of MRI to define potential biomarkers of NAFLD is emerging but not completely studied yet[73]. Proton density fat fraction is a consistent and easily reproducible marker of liver fat content. It serves as a non-invasive alternative to liver biopsy, currently the gold standard. An abbreviated protocol for liver fat estimation, along with or without liver iron content combined with MR elastography, may emerge as an effective screening tool for screening and quantification of NAFLD. Future research may include precise thresholds for abnormal liver fat.
Current MRI practice for the detection of HCC involves a complete abdominal MRI exam, which is time-consuming, expensive, and perhaps, less available worldwide, limiting its utility as a surveillance test. The emerging protocols for abbreviated MRIs or focused-guided exams have the potential to be used for surveillance in high-risk populations[21]. The examinations have the potential to be used for surveillance with the hope of being reproducible and cost-effective. At the current time, LIRADS needs to have optimized strategies, which will be addressed in further editions of the LIRADS document[9,11].
The United states LIRADS document does not provide definitive guidance on surveillance for HCC in patients with a visualization score of C on ultrasonography, which has a reduced sensitivity for HCC detection[46]. Future editions of LIRADS, in partnership with hepatology societies, need to develop further guidance for this particular category of patients, considering the potential utilization of abbreviated MRI surveillance.
Contrast-enhanced US, as an alternative for HCC surveillance, has the disadvantage of operator variability. The use in severe NAFLD decreases sensitivity, as these patients are typically difficult to image because of obesity and cirrhosis. There is a potential risk of misdiagnosis of ICCA and HCC. The advantages of CEUS include the ability to be used in patients with poor renal function, cost-effectiveness, and better availability, worldwide.
A cost-effectiveness analysis comparing contrast-enhanced MRI with US for HCC screening in patients with compensated cirrhosis provides initial encouraging results[74]. Despite the higher cost of MRI compared to US, MRI was a more cost-effective surveillance tool due to higher sensitivity in the detection of early HCC. The annual HCC incidence is the most influential factor. The estimated incremental cost‐effectiveness ratio (ICERs) are $10,721/QALY at an HCC incidence of 5% and $1,155/QALY at an HCC incidence of 10%, respectively. When the annual HCC incidence rate was greater than 1.81%, the ICER was below $50,000/QALY, which is the accepted cut-off point in the United States. Nonetheless, these encouraging results significantly lack generalizability. The main cause of liver disease in this Korean cohort was HBV infection, and, in fact, the authors only reported on HCC related to viral and alcoholic liver disease. Rates of obesity and NAFLD are on the rise in Korea; however, there are nowhere near the rates seen in the U.S.. It remains to be speculated if screening with contrast-enhanced MRI would be even more cost-effective in a cohort of patients with NAFLD/NASH liver disease.
Optimizing patient’ selection for HCC screening
There is a significant uptick in HCC in patients with underlying NAFLD/NASH fueled by the epidemic of obesity and metabolic syndrome associated with poor lifestyle choices[75]. The debate about screening all versus model-based screening strategies is ongoing. Given the large number of patients, universal screening, especially for those without advanced fibrosis or cirrhosis, is not feasible. Identifying high-risk patients is paramount and diagnosis and staging of liver fibrosis are crucial. We, therefore, suggest using patient-derived risk factors and developing a scoring system to stratify NAFLD/NASH patients into low and high-risk groups, which may aid in the decision-making to use MRI in addition to, or alternating with, regular screening ultrasonography. Ioannou et al. used data from a large Veterans Affairs cohort of patients with NAFLD-related cirrhosis and developed a risk score[76]. This patient-derived risk score includes age, gender, BMI, diabetes, platelet count, albumin, and AST to ALT ratio. The score separates patients into high (> 3%/year), intermediate (1-3%/year), and low risk (< 1%/year) of developing HCC, although the discrimination was just in the acceptable range (AUC 0.75).
A GALAD[Gender, Age, AFP-L3, AFP. Des-carboxy-prothrombin (DCP)] score of less than 0.63 was able to identify HCC in NAFLD[77]. A recently published case-control study performed in Germany as well as a pilot cohort study in Japan supports that the GALAD score can detect early-stage HCC, and subsequently may facilitate the screening of patients with NASH[78].
However, current hepatology guidelines do not recommend screening for HCC in patients with NAFLD-related liver disease based on a risk stratification score.
Several risk factors for NAFLD/NASH-related HCC have been established:
a) Family history of HCC as a known risk factor should be obtained.
b) BMI appears to be less important than body composition determining prognosis in HCC patients; however, the waist-to-hip ratio (WHR) has been identified as a promotor in HCC development and outcome[79,80]. Visceral adiposity was found to be linked to higher mortality in a large retrospective cohort study of 1,257 patients with HCC[81].
c) Another well-known key factor in HCC development and prognosis is insulin resistance (IR). In several case series and cohort studies, a strong link between IR and diabetes has been established[82-86]. Diabetes was confirmed as an independent risk factor for HCC development reported in 12 cohort studies[86].
d) The risk increases with age. Patients with NAFLD-related HCC are 5-10 years older at presentation compared to patients with HCC related to other liver disease[87].
e) Hepatic iron deposition is an established risk factor for HCC in NASH cirrhosis[88], and MRI can aid in iron quantification in identified patients.
f) Alcohol consumption increases the risk of HCC in patients with NAFLD[32], particularly with relatively heavy alcohol use (e.g., > 50 g/day).
g) Certain medications are associated with a decreased risk of HCC, including metformin[89],aspirin[90], and statins[91] while sulfonylureas and insulin are associated with an increased risk of HCC[91].
h) Last but not least, the patient's genetic background plays a role in NAFLD/NASH-related HCC. Genome-wide expression profiling has emerged to identify novel diagnostic and prognostic molecular biomarkers for many diseases including NAFLD and HCC. The patatin-like phospholipase 3 (PNPLA3) polymorphism has been identified to be strongly associated with NAFLD and NAFLD-HCC. The PNPLA3 G allele appears to be associated with the development and progression of NAFLD. The PNPLA3 genotype CC is significantly decreased in patients with NAFLD cirrhosis, while the KCNQ1 genotype TT is significantly increased in NAFLD-HCC. Furthermore, a strong association between the PNPLA3 G allele and NAFLD-HCC has been identified[86]. Considering all of the above, the stage of fibrosis is the strongest liver-related predictor of overall survival and risk of developing HCC in patients with NAFLD. Beyond the distinction between cirrhosis versus no cirrhosis, progressing fibrosis is also associated with increased HCC risk. The stage of fibrosis needs to be determined either by biopsy/histology or with non-invasive fibrosis tests such as elastography or serum fibrosis biomarker panels. Several available laboratory tests can be used as “predictors” of HCC, including low platelet count, high AST/ALT ratio, and low albumin.
Of concern is the development of HCC in patients without established cirrhosis. Subsequently, in these patients, we miss the opportunity to screen and detect early cancers, as they do not meet the criteria for screening, as recommended by professional societies. Contrast-enhanced aMRI techniques may emerge as future screening tools to identify at-risk patients and enhance early detection of HCC. This in turn will further improve outcomes. Prospective studies are needed to identify those high-risk patients, given the epidemic of obesity and NAFLD universal screening is impractical.
To date, MRI protocols for surveillance of lesions found in US and confirmation of HCC diagnosis include various contrast agents as well as different timing and sequence protocols depending on local preference. Further Characterization and standardization are needed to determine optimal imaging strategies in these patients.
In summary, after this current literature review, we do not find that HCC arising in NAFLD/NASH has particular or distinct features on MRI compared to other etiologies.
Future population-based studies are urgently needed to identify high-risk populations who would benefit from surveillance, including identifying the etiology and severity of liver disease, as well as blood, genetic, and imaging biomarkers.
Future studies should target the identification of high-risk patients who may potentially benefit from using MRI as a screening tool, and establish standardized protocols using optimal image sequencing and the most suitable contrast agents. More reliable and evidence-based data ideally obtained by randomized clinical trials are needed to support the development of professional guidelines addressing screening and surveillance for HCC for this growing at-risk population related to the epidemic of obesity, metabolic syndrome, and NAFLD/NASH-related liver disease.
DECLARATIONS
Authors’ contributionsConception and design of the manuscript: Pocha C, Choudhry S
Focused more on the clinical: Pocha C
Focused more on technical aspects: Choudhry S
Availability of data and materialsNot applicable.
Financial support and sponsorshipNot applicable.
Conflicts of interestNot applicable.
Ethical approval and consent to participateNot applicable.
Consent for publicationNot applicable.
Copyright© The Author(s) 2023.
REFERENCES
1. Charlton MR, Burns JM, Pedersen RA, Watt KD, Heimbach JK, Dierkhising RA. Frequency and outcomes of liver transplantation for nonalcoholic steatohepatitis in the United States. Gastroenterology 2011;141:1249-53.
2. Loomba R, Lim JK, Patton H, El-Serag HB. AGA clinical practice update on screening and surveillance for hepatocellular carcinoma in patients with nonalcoholic fatty liver disease: expert review. Gastroenterology 2020;158:1822-30.
3. Huang DQ, El-Serag HB, Loomba R. Global epidemiology of NAFLD-related HCC: trends, predictions, risk factors and prevention. Nat Rev Gastroenterol Hepatol 2021;18:223-38.
4. Leung C, Yeoh SW, Patrick D, et al. Characteristics of hepatocellular carcinoma in cirrhotic and non-cirrhotic non-alcoholic fatty liver disease. World J Gastroenterol 2015;21:1189-96.
5. Al-Sharhan F, Dohan A, Barat M, et al. MRI presentation of hepatocellular carcinoma in non-alcoholic steatohepatitis (NASH). Eur J Radiol 2019;119:108648.
6. Mikuriya Y, Tashiro H, Kobayashi T, et al. Clinicopathological features of hepatocellular carcinoma in patients with nonalcoholic fatty liver disease. Langenbecks Arch Surg 2015;400:471-6.
7. Asfari MM, Talal Sarmini M, Alomari M, Lopez R, Dasarathy S, McCullough AJ. The association of nonalcoholic steatohepatitis and hepatocellular carcinoma. Eur J Gastroenterol Hepatol 2020;32:1566-70.
8. Llovet JM, Castet F, Heikenwalder M, et al. Immunotherapies for hepatocellular carcinoma. Nat Rev Clin Oncol 2022;19:151-72.
9. Rizzo A, Ricci AD, Di Federico A, et al. Predictive biomarkers for checkpoint inhibitor-based immunotherapy in hepatocellular carcinoma: where do we stand? Front Oncol 2021;11:803133.
10. Finn RS, Qin S, Ikeda M, et al. IMbrave150 Investigators. Atezolizumab plus bevacizumab in unresectable hepatocellular carcinoma. N Engl J Med 2020;382:1894-905.
11. Lin ZF, Qin LX, Chen JH. Biomarkers for response to immunotherapy in hepatobiliary malignancies. Hepatobiliary Pancreat Dis Int 2022;21:413-9.
12. Gong XQ, Tao YY, Wu YK, et al. Progress of MRI radiomics in hepatocellular carcinoma. Front Oncol 2021;11:698373.
13. Yoon JK, Choi JY, Rhee H, Park YN. MRI features of histologic subtypes of hepatocellular carcinoma: correlation with histologic, genetic, and molecular biologic classification. Eur Radiol 2022;32:5119-33.
14. Marrero JA, Kulik LM, Sirlin CB, et al. Diagnosis, staging, and management of hepatocellular carcinoma: 2018 practice guidance by the American Association for the study of liver diseases. Hepatology 2018;68:723-50.
15. Association for the Study of the Liver. Electronic address: easloffice@easloffice.eu, European Association for the Study of the Liver. EASL clinical practice guidelines: management of hepatocellular carcinoma. J Hepatol 2018;69:182-236.
16. Patel K, Sebastiani G. Limitations of non-invasive tests for assessment of liver fibrosis. JHEP Rep 2020;2:100067.
17. Trépo E, Valenti L. Update on NAFLD genetics: from new variants to the clinic. J Hepatol 2020;72:1196-209.
18. Association for the Study of the Liver. Electronic address: easloffice@easloffice.eu, Clinical Practice Guideline Panel, Chair:, EASL Governing Board representative:, Panel members:. EASL clinical practice guidelines on non-invasive tests for evaluation of liver disease severity and prognosis - 2021 update. J Hepatol 2021;75:659-89.
19. Riley TR 3rd, Mendoza A, Bruno MA. Bedside ultrasound can predict nonalcoholic fatty liver disease in the hands of clinicians using a prototype image. Dig Dis Sci 2006;51:982-5.
20. Starekova J, Hernando D, Pickhardt PJ, Reeder SB. Quantification of liver fat content with CT and MRI: state of the art. Radiology 2021;301:250-62.
21. Pickhardt PJ. Abdominal imaging in the coming decades: better, faster, safer, and cheaper? Radiology 2023;307:e222551.
22. Huang DQ, Fowler KJ, Liau J, et al. Comparative efficacy of an optimal exam between ultrasound versus abbreviated MRI for HCC screening in NAFLD cirrhosis: a prospective study. Aliment Pharmacol Ther 2022;55:820-7.
23. Zhang J, Yu Y, Li Y, Wei L. Diagnostic value of contrast-enhanced ultrasound in hepatocellular carcinoma: a meta-analysis with evidence from 1998 to 2016. Oncotarget 2017;8:75418-26.
24. Available from: https://www.acr.org/Clinical-Resources/Reporting-and-Data-Systems/LI-RADS/LI-RADS-CEUS-v2017 [Last accessed on 24 May 2023].
25. Kono Y, Lyshchik A, Cosgrove D, et al. Contrast enhanced ultrasound (CEUS) Liver imaging reporting and data system (LI-RADS®): the official version by the American college of radiology (ACR). Ultraschall Med 2017;38:85-6.
26. Hu YX, Shen JX, Han J, et al. Diagnosis of non-hepatocellular carcinoma malignancies in patients with risks for hepatocellular carcinoma: CEUS LI-RADS versus CT/MRI LI-RADS. Front Oncol 2021;11:641195.
27. Schellhaas B, Jesper D, Strobel D. DEGUM CEUS HCC Study Group. Contrast-enhanced ultrasound pattern of hepatocellular carcinoma in noncirrhotic liver - results from the prospective multicentre DEGUM CEUS HCC study. Eur J Gastroenterol Hepatol 2023;35:313-9.
28. Bartolotta TV, Randazzo A, Bruno E, Taibbi A. Focal liver lesions in cirrhosis: role of contrast-enhanced ultrasonography. World J Radiol 2022;14:70-81.
29. Eisenbrey JR, Gabriel H, Savsani E, Lyshchik A. Contrast-enhanced ultrasound (CEUS) in HCC diagnosis and assessment of tumor response to locoregional therapies. Abdom Radiol 2021;46:3579-95.
30. Westwood M, Joore M, Grutters J, et al. Contrast-enhanced ultrasound using SonoVue® (sulphur hexafluoride microbubbles) compared with contrast-enhanced computed tomography and contrast-enhanced magnetic resonance imaging for the characterisation of focal liver lesions and detection of liver metastases: a systematic review and cost-effectiveness analysis. Health Technol Assess 2013;17:1-243.
31. Snowberger N, Chinnakotla S, Lepe RM, et al. Alpha fetoprotein, ultrasound, computerized tomography and magnetic resonance imaging for detection of hepatocellular carcinoma in patients with advanced cirrhosis. Aliment Pharmacol Ther 2007;26:1187-94.
32. Rode A, Bancel B, Douek P, et al. Small nodule detection in cirrhotic livers: evaluation with US, spiral CT, and MRI and correlation with pathologic examination of explanted liver. J Comput Assist Tomogr 2001;25:327-36.
33. Power SP, Moloney F, Twomey M, James K, O'Connor OJ, Maher MM. Computed tomography and patient risk: facts, perceptions and uncertainties. World J Radiol 2016;8:902-15.
34. Renzulli M, Golfieri R. Bologna Liver Oncology Group (BLOG). Proposal of a new diagnostic algorithm for hepatocellular carcinoma based on the Japanese guidelines but adapted to the Western world for patients under surveillance for chronic liver disease. J Gastroenterol Hepatol 2016;31:69-80.
35. Golfieri R, Garzillo G, Ascanio S, Renzulli M. Focal lesions in the cirrhotic liver: their pivotal role in gadoxetic acid-enhanced MRI and recognition by the Western guidelines. Dig Dis 2014;32:696-704.
36. Inoue T, Kudo M, Komuta M, et al. Assessment of Gd-EOB-DTPA-enhanced MRI for HCC and dysplastic nodules and comparison of detection sensitivity versus MDCT. J Gastroenterol 2012;47:1036-47.
37. Lee YJ, Lee JM, Lee JS, et al. Hepatocellular carcinoma: diagnostic performance of multidetector CT and MR imaging-a systematic review and meta-analysis. Radiology 2015;275:97-109.
38. Choi JY, Lee JM, Sirlin CB. CT and MR imaging diagnosis and staging of hepatocellular carcinoma: part II. Extracellular agents, hepatobiliary agents, and ancillary imaging features. Radiology 2014;273:30-50.
40. Pasquini L, Napolitano A, Visconti E, et al. Gadolinium-based contrast agent-related toxicities. CNS Drugs 2018;32:229-40.
41. Nahon P, Najean M, Layese R, et al. ANRS CO12 CirVir. Early hepatocellular carcinoma detection using magnetic resonance imaging is cost-effective in high-risk patients with cirrhosis. JHEP Rep 2022;4:100390.
42. Kim SY, An J, Lim YS, et al. MRI with liver-specific contrast for surveillance of patients with cirrhosis at high risk of hepatocellular carcinoma. JAMA Oncol 2017;3:456-63.
43. Kim HA, Kim KA, Choi JI, et al. Comparison of biannual ultrasonography and annual non-contrast liver magnetic resonance imaging as surveillance tools for hepatocellular carcinoma in patients with liver cirrhosis (MAGNUS-HCC): a study protocol. BMC Cancer 2017;17:877.
44. Cunha G, Chernyak V, Fowler KJ, Sirlin CB. Up-to-date role of CT/MRI LI-RADS in hepatocellular carcinoma. J Hepatocell Carcinoma 2021;8:513-27.
45. Liu JKJ, Lee CH, Tan CH. Evaluation of non-contrast magnetic resonance imaging as an imaging surveillance tool for hepatocellular carcinoma in at-risk patients. Singapore Med J 2022;63:203-8.
46. LIRADS R, v2018 CT/MRI Manual. Available from: https://www.acr.org/Clinical-Resources/Reporting-and-Data-Systems/LI-RADS/LI-RADS-CT-MRI-v2018 [Last accessed on 6 May 2023].
47. Davenport MS, Viglianti BL, Al-Hawary MM, et al. Comparison of acute transient dyspnea after intravenous administration of gadoxetate disodium and gadobenate dimeglumine: effect on arterial phase image quality. Radiology 2013;266:452-61.
48. Horowitz JM, Kamel IR, Arif-Tiwari H, et al. Expert panel on gastrointestinal imaging:. ACR appropriateness criteria(®) chronic liver disease. J Am Coll Radiol 2017;14:S103-17.
49. Chang SD, Cunha GM, Chernyak V. MR imaging contrast agents: role in imaging of chronic liver diseases. Magn Reson Imaging Clin N Am 2021;29:329-45.
50. Min JH, Kim JM, Kim YK, et al. Prospective intraindividual comparison of magnetic resonance imaging with gadoxetic acid and extracellular contrast for diagnosis of hepatocellular carcinomas using the liver imaging reporting and data system. Hepatology 2018;68:2254-66.
51. Liu X, Jiang H, Chen J, Zhou Y, Huang Z, Song B. Gadoxetic acid disodium-enhanced magnetic resonance imaging outperformed multidetector computed tomography in diagnosing small hepatocellular carcinoma: a meta-analysis. Liver Transpl 2017;23:1505-18.
52. Ueno A, Masugi Y, Yamazaki K, et al. OATP1B3 expression is strongly associated with Wnt/β-catenin signalling and represents the transporter of gadoxetic acid in hepatocellular carcinoma. J Hepatol 2014;61:1080-7.
54. Semelka RC, Brown ED, Ascher SM, et al. Hepatic hemangiomas: a multi-institutional study of appearance on T2-weighted and serial gadolinium-enhanced gradient-echo MR images. Radiology 1994;192:401-6.
55. Kang HJ, Kim H, Lee DH, et al. Gadoxetate-enhanced MRI features of proliferative hepatocellular carcinoma are prognostic after surgery. Radiology 2021;300:572-82.
56. Moon JY, Min JH, Kim YK, et al. Prognosis after curative resection of single hepatocellular carcinoma with a focus on LI-RADS targetoid appearance on preoperative gadoxetic acid-enhanced MRI. Korean J Radiol 2021;22:1786-96.
57. Kim SS, Lee S, Kim MJ. Prognostic factors of gadoxetic acid-enhanced MRI for postsurgical outcomes in multicentric hepatocellular carcinoma. Eur Radiol 2021;31:3405-16.
58. Motosugi U, Ichikawa T, Sou H, et al. Distinguishing hypervascular pseudolesions of the liver from hypervascular hepatocellular carcinomas with gadoxetic acid-enhanced MR imaging. Radiology 2010;256:151-8.
59. Davenport MS, Malyarenko DI, Pang Y, Hussain HK, Chenevert TL. Effect of gadoxetate disodium on arterial phase respiratory waveforms using a quantitative fast fourier transformation-based analysis. AJR Am J Roentgenol 2017;208:328-36.
60. Lanier H, Wallace A, Khanna G. Rate of gadoxetate disodium (Eovist®) induced transient respiratory motion in children and young adults. Abdom Radiol 2020;45:101-6.
61. Marks RM, Masch WR, Chernyak V. LI-RADS: past, present, and future, from the AJR special series on radiology reporting and data systems. AJR Am J Roentgenol 2021;216:295-304.
62. Elsayes KM, Kielar AZ, Chernyak V, et al. LI-RADS: a conceptual and historical review from its beginning to its recent integration into AASLD clinical practice guidance. J Hepatocell Carcinoma 2019;6:49-69.
63. Cunha GM, Sirlin CB, Fowler KJ. Imaging diagnosis of hepatocellular carcinoma: LI-RADS. Chin Clin Oncol 2021;10:3.
64. An C, Kim DY, Choi JY, Han KH, Roh YH, Kim MJ. Noncontrast magnetic resonance imaging versus ultrasonography for hepatocellular carcinoma surveillance (MIRACLE-HCC): study protocol for a prospective randomized trial. BMC Cancer 2018;18:915.
65. Available from: https://clinicaltrials.gov/ct2/show/NCT04539717[Last accessed on 24 May 2023].
66. Vietti Violi N, Lewis S, Liao J, et al. Gadoxetate-enhanced abbreviated MRI is highly accurate for hepatocellular carcinoma screening. Eur Radiol 2020;30:6003-13.
67. Chan MV, McDonald SJ, Ong YY, et al. HCC screening: assessment of an abbreviated non-contrast MRI protocol. Eur Radiol Exp 2019;3:49.
68. Kim DW, Choi SH, Kim SY, et al. Diagnostic performance of MRI for HCC according to contrast agent type: a systematic review and meta-analysis. Hepatol Int 2020;14:1009-22.
69. Roberts LR, Sirlin CB, Zaiem F, et al. Imaging for the diagnosis of hepatocellular carcinoma: a systematic review and meta-analysis. Hepatology 2018;67:401-21.
70. Liu YI, Shin LK, Jeffrey RB, Kamaya A. Quantitatively defining washout in hepatocellular carcinoma. AJR Am J Roentgenol 2013;200:84-9.
71. Barat M, Nguyen TTL, Hollande C, et al. LI-RADS v2018 major criteria: do hepatocellular carcinomas in non-alcoholic steatohepatitis differ from those in virus-induced chronic liver disease on MRI? Eur J Radiol 2021;138:109651.
72. Thompson SM, Garg I, Ehman EC, et al. Non-alcoholic fatty liver disease-associated hepatocellular carcinoma: effect of hepatic steatosis on major hepatocellular carcinoma features at MRI. Br J Radiol 2018;91:20180345.
73. Valenti L, Pedica F, Colombo M. Distinctive features of hepatocellular carcinoma in non-alcoholic fatty liver disease. Dig Liver Dis 2022;54:154-63.
74. Kim HL, An J, Park JA, et al. Magnetic resonance imaging is cost-effective for hepatocellular carcinoma surveillance in high-risk patients with cirrhosis. Hepatology 2019;69:1599-613.
75. Pocha C, Xie C. Hepatocellular carcinoma in alcoholic and non-alcoholic fatty liver disease-one of a kind or two different enemies? Transl Gastroenterol Hepatol 2019;4:p. 72.
76. Ioannou GN, Green P, Lowy E, Mun EJ, Berry K. Differences in hepatocellular carcinoma risk, predictors and trends over time according to etiology of cirrhosis. PLoS One 2018;13:e0204412.
77. Yang JD, Addissie BD, Mara KC, et al. GALAD score for hepatocellular carcinoma detection in comparison with liver ultrasound and proposal of GALADUS score. Cancer Epidemiol Biomarkers Prev 2019;28:531-8.
78. Best J, Bechmann LP, Sowa JP, et al. GALAD Score detects early hepatocellular carcinoma in an international cohort of patients with nonalcoholic steatohepatitis. Clin Gastroenterol Hepatol 2020;18:728-735.e4.
79. Ohki T, Tateishi R, Shiina S, et al. Visceral fat accumulation is an independent risk factor for hepatocellular carcinoma recurrence after curative treatment in patients with suspected NASH. Gut 2009;58:839-44.
80. Fujiwara N, Nakagawa H, Kudo Y, et al. Sarcopenia, intramuscular fat deposition, and visceral adiposity independently predict the outcomes of hepatocellular carcinoma. J Hepatol 2015;63:131-40.
81. Welzel TM, Graubard BI, Zeuzem S, et al. Metabolic syndrome increases the risk of primary liver cancer in the United States: a study in the SEER-Medicare database. Hepatology 2011;54:463-71.
82. Chen CL, Yang HI, Yang WS, et al. Metabolic factors and risk of hepatocellular carcinoma by chronic hepatitis B/C infection: a follow-up study in Taiwan. Gastroenterology 2008;135:111-21.
83. Wideroff L, Gridley G, Mellemkjaer L, et al. Cancer incidence in a population-based cohort of patients hospitalized with diabetes mellitus in Denmark. J Natl Cancer Inst 1997;89:1360-5.
84. Adami HO, Chow WH, Nyrén O, et al. Excess risk of primary liver cancer in patients with diabetes mellitus. J Natl Cancer Inst 1996;88:1472-7.
85. El-Serag HB, Hampel H, Javadi F. The association between diabetes and hepatocellular carcinoma: a systematic review of epidemiologic evidence. Clin Gastroenterol Hepatol 2006;4:369-80.
86. Rosmorduc O, Fartoux L. HCC and NASH: how strong is the clinical demonstration? Clin Res Hepatol Gastroenterol 2012;36:202-8.
87. Pais R, et al. , Temporal trends, clinical patterns and outcomes of NAFLD-related HCC in patients undergoing liver resection over a 20-year period. Aliment Pharmacol Ther 2017;46:p.856-63.
88. Sorrentino P, et al. Liver iron excess in patients with hepatocellular carcinoma developed on non-alcoholic steato-hepatitis. J Hepatol 2009;50:p.351-7.
89. Zhang H, Gao C, Fang L, Zhao HC, Yao SK. Metformin and reduced risk of hepatocellular carcinoma in diabetic patients: a meta-analysis. Scand J Gastroenterol 2013;48:78-87.
90. Tan JL, Sidhu-Brar S, Woodman R, Chinnaratha MA. Regular Aspirin Use Is Associated with a Reduced Risk of Hepatocellular Carcinoma (HCC) in Chronic Liver Disease: a Systematic Review and Meta-analysis. J Gastrointest Cancer 2022; doi: 10.1007/s12029-022-00842-y.
91. Goh MJ, Sinn DH. Statin and aspirin for chemoprevention of hepatocellular carcinoma: Time to use or wait further? Clin Mol Hepatol 2022;28:p.380-395.
92. Yamaoka K, Saitoh S, Kinowaki K, et al. Clinicopathological assessment of steatohepatitic hepatocellular carcinoma. Clin Res Hepatol Gastroenterol 2022;46:101799.
Cite This Article
Export citation file: BibTeX | RIS
OAE Style
Pocha C, Choudhry S. MRI for screening and surveillance for hepatocellular cancer in NAFLD and NASH. Hepatoma Res 2023;9:22. http://dx.doi.org/10.20517/2394-5079.2023.08
AMA Style
Pocha C, Choudhry S. MRI for screening and surveillance for hepatocellular cancer in NAFLD and NASH. Hepatoma Research. 2023; 9: 22. http://dx.doi.org/10.20517/2394-5079.2023.08
Chicago/Turabian Style
Pocha, Christine, Sabina Choudhry. 2023. "MRI for screening and surveillance for hepatocellular cancer in NAFLD and NASH" Hepatoma Research. 9: 22. http://dx.doi.org/10.20517/2394-5079.2023.08
ACS Style
Pocha, C.; Choudhry S. MRI for screening and surveillance for hepatocellular cancer in NAFLD and NASH. Hepatoma. Res. 2023, 9, 22. http://dx.doi.org/10.20517/2394-5079.2023.08
About This Article
Special Issue
Copyright
Data & Comments
Data
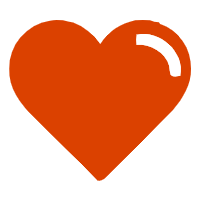

Comments
Comments must be written in English. Spam, offensive content, impersonation, and private information will not be permitted. If any comment is reported and identified as inappropriate content by OAE staff, the comment will be removed without notice. If you have any queries or need any help, please contact us at support@oaepublish.com.